The past 150 years have witnessed remarkable scientific, medical and technical improvements and advances. Many of these improvements and advances have impacted the study of the auditory system and treatment of disorders and diseases of that system.
In the late 19th Century, von Helmholtz (1862, 1885) conceived a sophisticated theory of hearing that invoked the presence of highly selective resonators in the cochlea. Approximately 50 years later, von Bekesy (1928, reprinted in von Bekesy, 1960) discovered the cochlear traveling wave phenomenon and fashioned a theory that disputed von Helmholtz' "resonance" theory. von Bekesy believed the ear could not contain enough individual resonators to cover the full range of pitch perception or that they could be tuned with sufficient sharpness to explain precipitous high-frequency hearing loss.
Although the traveling wave phenomenon discovered by von Bekesy is believed to be the key element in the cochlea's analysis of sound, the initial observations of von Bekesy revealed responses that were neither sharply tuned nor of sufficient sensitivity to respond to threshold levels of stimulation. The difficulty extrapolating from von Bekesy's observations to real-life listening situations can be appreciated when one considers that his observations were confined to either cadaver ears or to mechanical models constructed to replicate conditions encountered in cadaver ears.
The discrepancies between the contemporary traveling wave demonstration and the tuning and sensitivity of the ear, were clearly described by Thomas Gold some 20 years after von Bekesy's discovery. Gold suggested that parts of von Helmholtz' resonance theory were in keeping with the results of listening studies conducted with persons who had normal hearing (Gold, 1948). However, his ideas were not well accepted. Much effort was spent reconciling von Bekesy's findings with later psychoacoustical findings by developing hypotheses regarding the "sharpening" effects of the peripheral and central nervous system.
By the early 1970s, sophisticated investigations of cochlear mechanics in living ears at near-threshold stimulus intensities revealed that healthy systems were capable of better frequency resolution and threshold sensitivity than were predicted on the basis of von Bekesy's observations (Rhode, 1971).
Approximately 50 years after von Bekesy's discovery, David Kemp reported that sound energy produced by the ear could be detected in the ear canal (Kemp, 1978). Called otoacoustic emissions (OAEs), these sounds offer evidence that the ear contains a source of energy and that the energy may fuel the sharp tuning and exquisite threshold sensitivity von Bekesy was unable to see in his experiments. Soon after Kemp's discovery there appeared reports that cochlear outer hair cells (OHC) were capable of movement in response to electrical stimuli provided in vitro (Brownell, 1983). As the 1980s dawned, Hallowell Davis (1983) coined the phrase cochlear amplifier to describe the phenomenon in which the inner ear responds to and resolves near-threshold stimuli.
OAE: Status 2002
Although the puzzle is far from complete, the current thinking is that the OHCs deliver a "gain" over the energy present in the stimulus arriving at the stapes footplate of about 1000:1 (Ruggero & Rich, 1991; Ruggero, 1992). This amplification is reduced in ears that are damaged or temporarily compromised. To a large degree, sensorineural hearing loss is now interpreted as a loss of an amplifier system, rather than a loss of the actual sensory transducers.
Otoacoustic emissions are intimately related to the status of the cochlear amplifier and they provide the researcher and clinician with noninvasive tools to peer into the inner ear with an acoustic microscope. In the 25 years since their discovery, what lessons have they taught us?
As Kemp (2002) has noted, the full potential of OAEs in the clinic will probably not be realized for many years. Nonetheless OAEs have demonstrated value in several areas. The most frequent application of OAEs in clinical environments has been to screen for hearing loss in newborn or pediatric populations and to supplement behavioral evaluations of children or multiply-handicapped individuals.
OAE Descriptions and Characteristics:
OAEs may be characterized by the stimulus employed to elicit them, or by the mechanism that underlies the response. The broad descriptions that have been commonly accepted follow:
Spontaneous OAEs (SOAEs):
Narrow-band signals that occur in the absence of any known stimulus. SOAEs can be detected in the majority of persons with normal pure tone thresholds. SOAEs range in amplitude from the noise floor of the equipment to approximately 20 dB SPL and are absent in frequency regions associated with hearing loss greater than about 30 dB HL (Bright, 2002). SOAEs are normally detected by executing a spectral analysis on the sound recorded from the ear canal of a participant seated in a quiet test environment.
SOAEs can be synchronized to external stimuli and extracted as Synchronized Spontaneous Otoacoustic Emissions (SSOAEs) using time-averaging procedures commonly employed to detect small physiological signals, such as the auditory brain stem response, in the presence of background noise. Because they are not detected universally in normal hearing individuals and because they are idiosyncratic in terms of frequency distribution and amplitudes, SSOAEs have not found widespread use in the clinic. When SSOAEs are present one can conclude that the ear is functioning normally across the outer hair cells that respond to the frequencies revealed in the SSOAEs. When they are absent, no conclusion is possible about hearing status: thresholds could be normal or elevated through the audiometric test range.
Transient Evoked Otoacoustic Emissions (TEOAEs): TEOAEs, also called "Kemp Echos", follow a brief stimulus. The time delay between the stimulus and the response allows the examiner to isolate the response. TEOAEs detected from normal ears mirror the spectral properties of the stimulus (Glattke & Robinette, 2002). TEOAE measures have been widely reported for clinical studies ranging from newborn screening to ongoing monitoring of audiologic status, as might be used with chemotherapy patients. Examples of TEOAE responses obtained from infants are illustrated in Figure 1. Technical details regarding the derivation of the numerical measures displayed in the results have been provided by Glattke, Pafitis, Cummiskey and Herer (1995). Although no universal standard exists, the measures involved in the determination of whether or not a TEOAE is present are reproducibility and signal to noise ratio. The two types of results are related to each other and both have been employed to establish "pass" criteria for individuals undergoing screening. The examples in Figure 1 include a clear "pass" with robust reproducibility and signal to noise ratio values and a clear "refer" with low noise levels and poor reproducibility and signal to noise ratio results.

Fig. 1
Stimulus Frequency Otoacoustic Emissions (SFOAEs): During stimulation with continuous pure tones, the energy in the ear canal includes both the incident stimulus, sound reflected from the tympanic membrane and sound that mirrors energy leaking back from the cochlea. The contribution of the cochlea is a version of the stimulus that appears with a time (phase) delay (Kemp, 2002). SFOAEs are difficult to extract from the other signals present in the ear canal, and, as a result, have not found their way into routine clinical practice.
Distortion Production Otoacoustic Emissions (DPOAEs): Distortion products are low intensity tonal signals occur during stimulation of the ear, like SFOAEs. A common way to record DPOAEs is to present two continuous signals, called primary tones, and analyze the spectrum of the sound detected in the external ear canal. DPOAEs are relatively easy to extract because they appear at frequencies that can be predicted exactly from the frequencies of the primary tones (Lonsbury-Martin and Martin, 2002). The most robust distortion product (DP) occurs at a frequency of 2f1-f2 where f2 = approximately 1.2 x f1. This places the frequency of the DP about 1/2 octave below f2.
For example, the ear's response to primary tones at 2000 and 2400 Hz will produce a robust distortion product at 1600 Hz. In this example, 2000 Hz equals f1, and 2400 equals f2. Therefore, two times 2000 = 4000 Hz, and of course 4000 minus f2 (in this case 2400 Hz) equals 1600 Hz.
DPOAEs are audible to many listeners and were reported by Giuseppe Tartini in 1714. They can be heard in performances of Il trillo del Diavolo composed by Tartini in the 1700s (Spalding, 1953. )
DPOAE measures, have been investigated by many individuals with such diverse goals as identification of hearing loss and exploration of cochlear mechanics in patients with Meniere's syndrome.
Examples of TEOAE and DPOAE results obtained in the same individual are provided in Figure 2. The DPOAE results illustrate the amplitude of the 2f1-f2 component as the frequency of f2 is changed from below 1000 Hz to above 5000 Hz in steps of approximately 1/3rd octave. The level of the noise in the plus 1 or 2 standard deviations in the recording is illustrated by the solid and cross-hatched areas, respectively. As in the case of TEOAEs, there is no universal standard for determining if DPOAEs are present in a recording. Some individuals report the signal-to-noise ratio of the DPOAE at various frequencies. Others rely on absolute amplitude measurements.
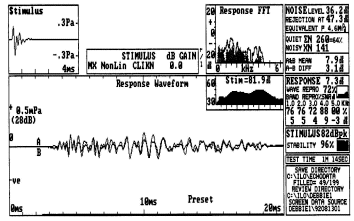

Fig. 2
Popular myths:
Two popular myths have made their way into current thinking. The first myth is that the DPOAE technique produces results that have more frequency specificity than TEOAE methods. The second myth is that DPOAEs are more robust than TEOAEs and, therefore, can be obtained under more challenging recording conditions. Neither of these assertions is correct when proper controls are in place.
As mentioned previously, TEOAEs mirror the spectrum of the stimulus. If one employs a click stimulus, then the TEOAE spectrum will be that of the click, or broad band. If a tone burst is used to elicit a TEOAE, then the response will mirror the frequency composition of the tone. Discrepancies between the stimulus and response spectra should raise suspicion that there are frequency-specific problems in the peripheral auditory system. In some instances the discrepancies may be due to acoustic properties of the recording system, but in many cases, they reflect peripheral auditory disorders.
The myth about the relative amplitudes of the DPOAE result and the TEOAE result stems from the intensity and complexity of the stimuli. The TEOAE stimulus typically is presented at an overall level of 80 dB peak SPL. Because the energy in the stimulus is spread over a broad frequency range, the energy at any individual frequency is about 35 dB below the overall level. That is to say, the spectrum level of the energy in the transient stimulus is about 35 dB below the overall level. The stimulus, therefore, is at about 40-45 dB SPL for any specific frequency.
In contrast, the primary tones used to elicit DPOAE results are presented at stimulus levels at 50 to about 70 dB SPL, depending on the interest of the examiner. If the DPOAE is more robust than the TEOAE in a given recording situation, one reason can be the difference in stimulus SPL. In Figure 2, the TEOAE response at individual frequencies is between -10 and - 5 dB SPL, or about 40 to 50 dB below the energy corresponding to those frequencies in the stimulus. The DPOAE response is about +5 to +10 dB SPL at individual frequencies, or about 50 to 60 dB below the SPL of the primary stimuli used to elicit the DPOAE.
Said another way, the salient features of the responses are determined not by some inherent difference in the TEOAEs and DPOAEs, but by the stimuli used to elicit them.
Screening for Hearing Loss:
TEOAEs have been employed in large-scale newborn hearing screening programs for more than 10 years. Although there are important exceptions, the majority of sites reporting data have used some version of the Otodynamics ILO hardware and software system to conduct hearing screenings.
Prieve (2002) recently summarized the results of 17 investigations involving more than 77,000 infants, all of whom were screened as inpatients. As her review indicated, criteria for "pass" and "refer" vary considerably. Some investigators use the "whole reproducibility" result. Others employ either reproducibility or signal-to-noise ratio criteria for individual frequency bands that are submitted to analysis. Other choices include the overall amplitude of the TEOAE and combinations of amplitude and reproducibility measurements.
Relatively few investigations have focused on the use of DPOAEs to screen newborn infants for hearing loss (Prieve, 2002) and "pass" versus "refer" criteria are not uniform among investigators (Gorga, Neely & Dorn, 2002). Some have employed absolute SPL of the DPOAE, while others have accepted signal to noise ratio measurements.
Sensitivity and Specificity Issues:
A major problem with the majority of investigations of newborn screening programs is the lack sensitivity and specificity data.
One reason for the lack of data is that babies who pass screens are rarely tracked long enough to determine if the "pass" was accurate. If some of the infants who pass the screen have hearing loss, then both sensitivity and specificity will suffer. In addition, the "gold standard" for newborn "hearing thresholds" is not a hearing test, but, rather, the auditory brain stem response (ABR). Reliable behavioral audiometry usually cannot be achieved until several months after birth.
Some information about the sensitivity and specificity of newborn hearing screening programs can be inferred from studies in mixed populations that compare audiometric results with OAE results.
For example, Gorga, Neely, Bergman, et al (1993), Pafitis, Cummiskey, Herer & Glattke (1994), Glattke, Pafitis, Cummiskey & Herer (1995), Dorn, Piskorski, Gorga, Neely, & Keefe (1999) have all demonstrated that there is an overlap in the distribution of results from normal and hearing impaired individuals. This means that neither sensitivity nor specificity can be perfect.
Commonly, sensitivity has been estimated at 85-95% and specificity at 90% or greater. This means that a small percentage of individuals with hearing loss can escape detection if OAEs are used to screen them. Similarly, a small percentage of persons with normal hearing thresholds for pure tones will fail the screen. Investigations that have demonstrated perfect sensitivity of OAE measures for hearing loss have employed relatively small samples and multiple OAE stimulus intensities (e.g., Harrison & Norton, 1999).
Additional information about newborns has come from three investigations that targeted infants for follow-up. All of these investigations followed children who ultimately provided behavioral audiometric test results. The behavioral tests usually involved visual reinforcement audiometry and were conducted several months after the initial OAE or other screening procedures. Lutman, Davis, Fortnum and Wood (1997) reported that the sensitivity of the OAE screening was between 80 and 90% for children ultimately confirmed with hearing loss by behavioral methods.
Mason, Davis, Wood and Farnsworth (1998) found similar results for ABR screening techniques. Finally, Norton, et al. (2000) report nearly identical findings not only for TEOAE, and DPOAE but also for ABR screening techniques. The investigations of Lutman, Mason, Norton and their co-workers are very important because they provide a realistic basis on which to build expectations regarding the outcome of hearing screening programs.
Specifically, they demonstrate that the OAE and ABR screening techniques have similar sensitivity when evaluated in targeted populations. Nonetheless, it is possible that some children not identified during the initial screening procedures acquired hearing loss in the first months of life. For instance, some children with CMV (cytomegalovirus) may pass the initial hearing screening, however, their hearing loss may occur after the screening, but before behavioral tests were completed. However, the similarity between the infant data and the results from studies of older patients is very clear.
One needs to remember that no physiological test is actually a test of hearing. OAEs provide information about the status of the auditory periphery, including the outer, middle and the "cochlear amplifier." There is also the possibility that OAE screening is insensitive to some types of disorders (e.g., neurological) that create impairment of hearing. Similarly ABR measures may not be sensitive to changes in middle ear status that can affect OAE results.
Universal screening programs based on physiological techniques have been the foundation for a revolution in screening activities that will help us move toward the goal of early detection of hearing loss, which was articulated by the NIH Consensus Conference (1993), the American Academy of Pediatrics (1999) and the Joint Committee on Infant Hearing (2000). As Kemp (2002) has suggested, it would be unfortunate if we were to abandon the search for understanding the information obtained in OAE recordings by arresting our clinical techniques at their present state of development.
Peering into the future:
There are many clinical objectives that might be reached through the use of OAE recordings. The first involves screening of populations other than newborns and infants (Nozza, 2001). As cited previously, there is a growing data base constructed from studies involving mixed populations of children and adults with audiometric and OAE data. This collective experience suggests that OAEs can be used across the life span to screen for the presence of hearing loss with excellent sensitivity and specificity. The data support the use of OAE measures to screen for hearing loss in virtually any group of patients who are unable to cooperate for behavioral audiometry.
The future need not be limited to screening for hearing loss, however.
For example, Sakashita and co-workers (1991) demonstrated that recovery from idiopathic sudden hearing loss may be associated with the presence of OAEs. Harris and Probst (1992) have examined patients with Meniere's syndrome and determined that OAEs may provide useful diagnostic information. Several investigators are exploring the relationships between OAEs and damage due to ototoxic medications and to noise exposure (e.g., Prasher & Sulkowski, 1999; Lonsbury-Martin & Martin, 2001). In addition, it is well known that OAEs may be altered by the presentation of ipsilateral or contralateral stimulation (e.g., Hood, 2002) presumably through the effects of efferent control over the action of cochlear outer hair cells. This OAE feedback system parallels the loop involved in the production of acoustic reflexes and offers the audiologist another tool with which to probe the integrity of the auditory system.
This is a very interesting time to be involved in the assessment and rehabilitation of persons with hearing loss. OAEs provide us with sensitive peeks into the function of the auditory periphery. Thus far, only a small portion of OAE potential has been tapped, primarily by efforts to use OAEs to screen for hearing loss. As Kemp (2002) has noted, OAEs continue to attract the keen interest of many researchers and, as a consequence, the clinical applications of OAEs are bound to prosper.
References:
American Academy of Pediatrics Task Force on Newborn and Infant Hearing (1999). Newborn and infant hearing loss: detection and intervention. Pediatrics, 103, 527-529.
Bright, K.E. (2002). Spontaneous otoacoustic emissions. in M.S.Robinette & T. J. Glattke (eds). Otoacoustic Emissions: Clinical Applications. (2nd ed) (pp 74-94). New York: Thieme.
Brownell, W.E. (1983). Observations on on motile response in isolated outer hair cells. in W. Webster & L. Aitkin, (eds). Mechanisms of Hearing. (pp 5-10). Clayton, Australia: Monash University Press.
von Bekesy, G. (1960). Experiments in Hearing. New York: McGraw Hill.
Davis, H. (1983). An active process in cochlear mechanics. Hearing Research, 9, 79-90.
Glattke, T.J. & Robinette, M.S. (2002). Transient evoked otoacoustic emissions. in M.S.Robinette & T. J. Glattke (eds). Otoacoustic Emissions: Clinical Applications. (2nd ed) (pp 95-115). New York: Thieme.
Gold, T. (1948). Hearing II. The physical basis of the action of the cochlea. Proceedings of the Royal Society (London), 135, 492-498.
Gorga, M.P. Neely, S.T., Bergman, B., et al. (1993). A coparison of transient evoked and distortion product otoacoustic emissions in normal hearing and hearing impaired subjects: Distortion product responses. Journal of the Acoustical Society of America, 94, 2639-2648.
Gorga, M.P. Neely, S.T. & Dorn, P.A. (2002). Distortion product otoacoustic emissions in relation to hearing loss. in M.S.Robinette & T. J. Glattke (eds). Otoacoustic Emissions: Clinical Applications. (2nd ed) (pp 243-272). New York: Thieme.
Harris, F.P., & Probst, R. (1992). Transiently evoked otoacoustic emissions in patients with Meniere's disease. Acta-Otolaryngologica, 112, 36-44.
Harrison, W.A., & Norton, S.J. (1999). Characteristics of transient evoked otoacoustic emissions in normal hearing and hearing impaired children. Ear and Hearing, 20, 75-86.
Hood, L.J. (2002). Suppression of otoacosustic emissions in normal individuals and in patients with auditory disorders. in M.S.Robinette & T. J. Glattke (eds). Otoacoustic Emissions: Clinical Applications. (2nd ed) (pp 325-347). New York: Thieme.
Joint Committee on Infant Hearing (2000). Position Statement.
Kemp, D.T. (1978). Stimulated acoustic emissions from within the human auditory system. Journal of the Acoustical Society of America, 64, 1386-1391.
Kemp, D.T. (2002). Exploring cochlear status with otoacoustic emissions. The potential for new clinical applications. in M.S.Robinette & T. J. Glattke (eds). Otoacoustic Emissions: Clinical Applications. (2nd ed) (pp 1-47). New York: Thieme.
Lonsbury-Martin, B.L., & Martin, G.K. (2002). Distortion product otoacoustic emissions in M.S.Robinette & T. J. Glattke (eds). Otoacoustic Emissions: Clinical Applications. (2nd ed) (pp 116-142). New York: Thieme.
Lutman, M., Davis, A.C., Fortnum, H.M., & Wood, S. (1997). Field sensitivity of targeted neonatal hearing screening by transient evoked otoacoustic emissions, Ear and Hearing, 18, 265-276.
Lonsbury-Martin, B.L., & Martin, G.K. (2001). Evoked otoacoustic emissions as objective screeners for ototoxicity. Seminars in Hearing, 22 (4), 377-391.
Mason, S., Davis, A., Wood, S. & Fansworth, A. (1998). Field sensitivity of targeted neonatal hearing screening using Nottingham ABR screener. Ear and Hearing, 19, 91-102.
National Institutes of Health (1993). Early identification of hearing impairment in infants and young children. NIH Consensus Statement. pp11-24.
Norton, S.J., Gorga, M.P., Widen, J.E., Folsom, R.C., Sininger, Y., Cone-Wesson, B., Vohr, B.R., Mascher, K., & Fletcher, K. (2000). Identification of neonatal hearing impairment: Evaluation of transient evoked otoacoustic emission, distortion product otoacoustic emission, and auditory brain stem response test performance. Ear and Hearing, 21, 508-528.
Nozza, R.J. (2001). Screening with otoacoustic emissions beyond the newborn period. Seminars in Hearing, 22 (4), 415-425.
Pafitis, I., Cummiskey, C., Herer, G., & Glattke, T. J. (1994). Detection of hearing loss using otoacoustic emissions. Proceedings of Internoise 94 (pp 763-768). Osaka: Institute of Noise Control Engineering/Japan & Acoustical Society of Japan.
Prasher, D., & Sulkowski, W. (1999). The role of otoacoustic emissions in screening and evaluation of noise damage. International journal of occupational medicine and environmental health, 12, 183-192.
Prieve, B. (2002). Otoacoustic emissions in neonatal hearing screening. in M.S.Robinette & T. J. Glattke (eds). Otoacoustic Emissions: Clinical Applications. (2nd ed) (pp 348-374). New York: Thieme
Rhode, W.S. (1971). Observations of the vibrations of the basilar membrane in squirrel monkeys using the Mossbauer technique. Journal of the Acoustical Society of America, 49, 1218-1231.
Ruggero, M.A. (1992). Responses to sound of the basilar membrane of the mammalian cochlea. Current Opinion in Neurobiology. 2(4):449-456.
Ruggero, M.A., & Rich, N.C. (1991). application of a commercially manufactured Doppler shift laster velocimeter to the measurement of basilar membrane vibration. Hearing Research, 51, 215-230.
Sakashita, T., Minowa, Y., Hachikawa, K., Kubo, T., Kakai, Y. (1991). Evoked otoacoustic emissions from ears with idiopathic sudden deafness. Acta-oto-laryngologica supplement. 48. 666-672.
Spalding, Albert (1953). A Fiddle, a Sword and a Lady. The Romance of Giuseppe Tartini. New York: Holt.