From the Desk of Gus Mueller
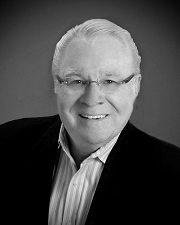
As with most professions, there are a lot of sub-specialties in audiology. Some of you focus almost entirely on adult diagnostics: air, bone, word recognition, immittance and OAEs and you pretty much can call it a day. It’s pretty common, however, to also include hearing aid sales and service, which adds a lot of extra things to think about. And, there of course is vestibular testing. And pediatrics. And, maybe even assessment of central auditory processing.
Regardless of what your typical workday looks like, there is one area where we all need to stay up-to-date—cochlear implants. We owe it to our patients to recognize candidacy, and provide them with the most current information regarding potential benefit and satisfaction.
Some of you might recall the great 20Q on cochlear implants for children written by Jace Wolfe last year, where he highlights research that examines the relationship between wear time and outcomes, and reviews the many factors that influence wear time. This was a follow-up to his earlier 20Q, sub-titled “Shooting for the Moon,” where he discusses some of the amazing outcomes observed today for children with cochlear implants.
There of course is a lot of new research findings in the area of cochlear implants for adults too. It sure would be great to read a review of all this. If only we knew someone who had published over 60 articles on this topic in the past five years. Oh wait . . . we do, and she is here this month to summarize some of her findings (and those of other experts too).
Margaret Dillon, AuD, PhD, is an Associate Professor in the Department of Otolaryngology/Head and Neck Surgery (OHNS) at the University of North Carolina (UNC) at Chapel Hill School of Medicine. She is the Director of OHNS Clinical Research and the Director of the Cochlear Implant Clinical Research Laboratories at UNC. She also is the Course Director for Advanced Signal Coding, a course for doctorate of audiology students.
Dr. Dillon’s research in the area of cochlear implants is extensive, in particular, studying new indications for cochlear implantation and methods to personalize the fitting and mapping of cochlear implant and electric-acoustic stimulation devices. She and co-authors were recently the recipients of an Ear & Hearing Readers’ Choice Award for their paper on guidelines for the clinical assessment and management of adult cochlear implantation for single-sided deafness.
Meg is a North Carolina native, who certainly has stayed true to her Tar Heel roots. Her Bachelors, AuD, and PhD all were obtained at UNC Chapel Hill. Moreover, her professional work history all has been at the UNC Chapel Hill Department of OHNS, going back to 2009.
Advances in this sub-specialty always are fascinating, and Meg does a great job of bringing us up-to-date in many areas of the audiology world of cochlear implants.
Gus Mueller, PhD
Contributing Editor
Browse the complete collection of 20Q with Gus Mueller CEU articles at www.audiologyonline.com/20Q
20Q: Update on Cochlear Implants: Hearing Preservation, Single-Sided Deafness, and Personalized Fitting
Learning Outcomes
After reading this article, professionals will be able to:
- List the new indications for cochlear implantation, including single-sided deafness.
- Discuss the benefits of cochlear implant use that have been reported for children and adults with single-sided deafness.
- Discuss the influence of electric frequency-to-place mismatches on speech recognition for cochlear implant and electric-acoustic stimulation device users, and methods to personalize the map to eliminate electric mismatches.
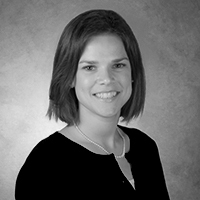
1. I’m so glad we are having this conversation. I don’t work directly with cochlear implants, but I know there are a lot of new things I need to know about. Where do we start?
Good question. I think we should start with the expansions in candidacy criteria for cochlear implantation. Today, cochlear implantation is a treatment option not only for patients with bilateral severe-to-profound hearing loss. Candidacy criteria now include patients with normal-to-moderate, low-to-mid frequency hearing thresholds in the ear to be implanted and also, patients with normal-to-moderate hearing thresholds in the contralateral ear. This includes cases of single-sided deafness (SSD).
These expansions in candidacy criteria make it challenging to know when to refer a patient for a cochlear implantation candidacy evaluation. Confusion regarding candidacy could be one reason why rates of cochlear implantation for qualified children and adults remain low (Marinelli et al., 2022; Nassiri et al., 2022; Sorkin, 2013). To help with this, new guidelines have been created for when to refer. Teresa Zwolan and colleagues (2020) discussed a 60/60 guideline for when to refer adult patients who may meet traditional indications for cochlear implantation. They recommended referring adults with a better ear pure-tone average (PTA: .5, 1, & 2 kHz) of 60 dB HL or poorer, and a better ear unaided monosyllabic word recognition score of 60% or less. For children, Lisa Park and colleagues (2021) recommended referring for a cochlear implantation evaluation for those with a PTA of 65 dB HL or poorer, an aided word recognition score of 60% or less, or an aided speech intelligibility index (SII) of 0.65 or poorer in at least one ear. Victoria Sweeney and colleagues (2022) also recommended referring patients with unilateral moderate-to-profound hearing loss and limited speech recognition in the affected ear – which would include children and adults with SSD.
Taken together, children and adults with better hearing in the ear-to-be implanted and in the contralateral ear are being considered for cochlear implantation than they were a few years ago due to the observed benefits of cochlear implant (CI) use for such cases in previous research.
Another important point is that there is no upper age limit for cochlear implantation. The cochlear implantation candidacy evaluation considers the patient’s overall health as opposed to age.
2. You mentioned two types of patient groups: (1) those with better hearing in the ear-to-be implanted and (2) those with better hearing in the contralateral ear. Let’s start with the first group.
For patients with normal-to-moderate low-to-mid frequency hearing thresholds and a severe-to-profound high-frequency hearing loss, CI teams are thinking about hearing preservation and how to support the best outcomes for these patients over time. One variable to consider is the length of the electrode array that is implanted. Traditionally, short (e.g., ≤ 24 mm) electrode arrays have been preferred for these patients because it was thought that they would induce the least amount of trauma to the functional hearing region of the cochlea (Gantz et al., 2016; Suhling et al., 2016; Wanna et al., 2018). A consideration of short electrode arrays is that if the patient loses functional acoustic hearing in the implanted ear, performance with a CI-alone device may be poorer compared to CI users with longer electrode arrays (Büchner et al., 2017). One reason for this could be that recipients of shorter electrode arrays listening with default CI maps will have larger spectral shifts in the electric frequency information relative to the cochlear place frequencies (also known as frequency-to-place mismatches) than recipients of longer electrode arrays. Interestingly, hearing preservation has also been reported for recipients of longer (e.g., 31.5 mm) lateral wall electrode arrays (Helbig et al., 2011; Hollis et al., 2021; Mick et al., 2014). For example, Emily Hollis and colleagues (2021) reported that 84% of their sample of CI recipients of a flexible, 31.5 mm lateral wall electrode array had functional hearing preservation (defined as ≤80 dB HL). It remains unclear what variables contribute to long-term hearing preservation (Jensen et al., 2022). Thus, CI teams are considering the likelihood of functional acoustic hearing preservation and the ability to support long-term success with the device when selecting the specific electrode array for these patients.
To take it a step further – hearing preservation has also been observed in patients who have undergone revision cochlear implantation (Helbig et al., 2013; Thompson et al., 2019). For example, Nicholas Thompson and colleagues (2019) reviewed the outcomes for a patient with normal low-frequency hearing thresholds whose internal device failed after ~10 years. The patient experienced similar hearing preservation post-reimplantation and continued to show a speech recognition benefit with the combination of his natural low-frequency acoustic hearing and the CI.
The punchline here is that hearing preservation is achievable for many patients and the field is continuing to investigate what contributes to hearing preservation both initially after surgery and over time.
Another consideration is that it is unclear how much residual hearing is needed to benefit from electric-acoustic stimulation (EAS). EAS is a term that we use to describe the listening condition of acoustic amplification (or natural hearing for patients with normal unaided low-frequency thresholds in the implanted ear) and a CI in the same ear. An EAS device has acoustic amplification and CI technologies combined in a single device. Data from bimodal listeners (CI in one ear and acoustic hearing/a hearing aid in the contralateral ear) demonstrate a benefit for speech recognition with the addition of 125 Hz (Zhang, Dorman, & Spahr, 2010) or 250 Hz (Sheffield & Gifford, 2014) low-passed acoustic signal to the electric stimulation. Our CI team currently recommends the fitting of an EAS device for patients with an unaided threshold in the implanted ear of 65 dB HL or better at 125 Hz.
3. How is the programming of an EAS device different from a CI-alone device?
With a CI-alone device, the default mapping procedure distributes the frequency information for the incoming signal across the active electrode channels. With an EAS device, the default mapping procedure divides that frequency information into what can be effectively represented acoustically and what should be represented electrically. The typical procedure is to first identify the acoustic cutoff, which is the highest frequency that we can aid the low frequencies. The acoustic cutoff frequency varies in definition but is usually the frequency at which the unaided hearing threshold exceeds 65 or 70 dB HL. It is important to verify the acoustic output since the settings for the acoustic component significantly affect the speech recognition of EAS users (Dillon et al., 2014). The acoustic cutoff frequency is then used as the low-frequency filter for the electric component, and the remaining frequency information is distributed across the active electrode channels. An aim of the EAS default mapping procedure is to provide the patient with the full speech spectrum – that is, it avoids gaps in the frequency information presented acoustically versus electrically.
Considering this, it is important to evaluate the unaided hearing thresholds in the implanted ear at follow-up visits to verify the acoustic output and assess whether the acoustic cutoff frequency should change. The default mapping procedure will change the electric filter frequencies when the acoustic cutoff frequency changes. This is important to consider when comparing benefit over time, since the patient likely will need time to acclimate to the new electric filter frequency assignments.
4. Are there recommendations for when to measure unaided hearing thresholds?
Considering fluctuations in acoustic hearing post-operatively, our clinical protocol is to measure unaided thresholds at the activation visit for CI recipients who presented with an unaided threshold of 80 dB HL or better at the preoperative visit (Dillon et al., 2020). For those with hearing preservation, unaided thresholds are measured at each follow-up visit to monitor for changes in acoustic hearing and for the fitting and verification of the acoustic component of the EAS device. If hearing preservation is not observed at the activation visit, unaided thresholds are measured at the 6 and 12 month visits to evaluate whether detection has improved and would warrant EAS fitting.
5. What are the benefits of listening with an EAS device as compared to a CI-alone device?
There is considerable evidence that listening with EAS as compared to a CI-alone supports better performance on measures of speech recognition in challenging background noise and sound source localization (Adunka et al., 2013; Dunn et al., 2010; Gantz et al., 2016; Gifford et al., 2013, 2014; Helbig, Van de Heyning, et al., 2011; Pillsbury et al., 2018). Patients listening with EAS also demonstrate better music perception than CI users (Gfeller et al., 2006). Additionally, patients perceive a greater benefit with EAS as compared to preoperative abilities with a hearing aid (Dillon et al., 2017; Gantz et al., 2016; Gstoettner et al., 2008). There is still variability in outcomes – but these data show that CI recipients benefit from the combination of acoustic and electric stimulation in the same ear.
6. Let’s switch to the other patient group you mentioned: those with relatively good hearing in the contralateral ear. When did this population become eligible for cochlear implantation, and what are the criteria for implantation?
In 2019, the FDA approved the expansion of candidacy criteria for cochlear implantation to include patients 5 years of age and older with profound sensorineural hearing loss in one ear and either normal hearing (referred to as single-sided deafness, or SSD) or mild to moderately-severe hearing loss (asymmetric hearing loss; AHL) in the other ear. This was exciting because we now evaluate the abilities of each ear independently and determine the appropriate treatment option so that the auditory inputs from each ear work well together.
7. Are there proven benefits of CI use for patients with SSD?
There have been multiple studies demonstrating the benefits of CI use for adults with SSD as compared to listening with the normal-hearing ear alone or when listening with a rerouting technology, such as a CROS hearing aid, or bone conduction devices (Arndt et al., 2011; Deep et al., 2021; Firszt et al., 2012; Galvin et al., 2019; Távora-Vieira et al., 2015; Van de Heyning et al., 2008). For example, adults with SSD experienced significant improvements with CI use on measures of speech recognition in noise (Buss et al., 2018) and sound source localization (Dillon, Buss, Anderson, et al., 2017), and reported significant improvements in quality of life (Dillon, Buss, Rooth, et al., 2017) as compared to preoperative abilities in our 12-month prospective clinical trial. Our team has seen that these performance benefits are either maintained or continue to grow significantly with long-term (>12 months) CI use (Dillon, Rooth, et al., 2022; Thompson, Brown, et al., 2022; Thompson, Dillon, et al., 2022; Thompson et al., 2023).
These findings are encouraging because they demonstrate the effectiveness of cochlear implantation and CI use for adults with SSD as compared to listening with the normal hearing ear alone or with alternative treatment options – which challenges what used to be best practice for this patient population.
8. You said earlier that the candidacy criteria now includes patients 5 years of age and up. What is known about CI use for pediatric cases of SSD?
The data from studies of CI use for children with SSD also demonstrate significant improvements on measures of speech recognition and sound source localization (Arndt et al., 2015; Benchetrit et al., 2021; Brown, Dillon, & Park, 2022; Ehrmann-Mueller et al., 2020; Park et al., 2023; Sladen et al., 2017; Távora-Vieira & Rajan, 2015; Thomas et al., 2017; Zeitler et al., 2019). For example, Kevin Brown and colleagues (2022) reported the outcomes of 20 children with SSD who participated in a prospective, repeated-measures clinical trial investigating outcomes of CI use as compared to listening with the normal-hearing ear alone. This study – known as the Children with Unilateral sensory Hearing Loss (CUHL) study – found that preschool and school-aged children with SSD experienced significant improvements on CNC word recognition with the CI when tested monaurally in their poorer (implanted) ear. Performance in the best-aided condition (CI plus the normal-hearing ear; CI+NH) also improved for speech recognition in spatially-separated noise and sound source localization during the first 12 months of CI use. Consistent with these behavioral outcome measures, parents of pediatric CI users report significant improvements in perceived abilities for their children when listening with the CI as compared to preoperative abilities (Beck et al., 2017; Brown et al., 2022; Lopez et al., 2021; Ramos Macías et al., 2019).
9. Are there different considerations for patients with SSD as compared to other patient populations?
From the current evidence, we think there are additional management considerations for patients with SSD as compared to patients with bilateral hearing loss. Two American Cochlear Implant Alliance (ACIA) Task Forces recently reviewed the current evidence of adult and pediatric CI users to generate guidelines for the clinical assessment and management of cochlear implantation for these patient populations (Dillon, Kocharyan, et al., 2022; Park et al., 2022). These guidelines were endorsed by the American Academy of Audiology.
Let’s start with some highlights of the guidelines from the team that reviewed the current evidence for adults (Dillon, Kocharyan, et al., 2022). For adults with acquired SSD, a 3-6 month monitoring period is recommended to see if patients with sudden hearing loss recover hearing; imaging is also recommended. It is unclear whether the duration of the hearing loss significantly influences outcomes for adults with acquired SSD. The research is limited for adults with congenital SSD, though data suggests that cortical reorganization in adults with long-term SSD may limit performance with the CI. Similar to studies of adult CI users with bilateral hearing loss, the research is mixed as to whether older age at implantation negatively influences outcomes for CI users with SSD. Importantly, while performance may be poorer for older adults than for younger adults – older adults with SSD have shown significant improvements with CI use. Also, counseling should include a discussion of non-surgical options for SSD (conventional amplification, bone conduction devices, or CROS/BiCROS hearing aids), the potential influence of cochlear implantation and CI use on tinnitus, and the need for consistent CI use.
Now switching to children, Lisa Park and colleagues (2022) reviewed the evidence to support guidelines for the clinical assessment and management of for this population with SSD. The integrity of the auditory nerve is a major consideration. A high percentage of children with SSD have cochlear nerve deficiency (CND), which the authors recommended as a contraindication for cochlear implantation. For example, Teresa Vos and colleagues (2022) reviewed the magnetic resonance imaging (MRI) for 204 pediatric patients with SSD and found evidence of CND in ~30% of the sample – which could not have been consistently determined with unaided hearing thresholds since some patients had measurable hearing. Considering this, high resolution MRI is recommended for children with congenital SSD. The influence of age at implantation and duration of SSD on outcomes with a CI are unclear. We might expect that younger age at implantation and shorter durations of SSD would be associated with better outcomes; however, research is needed. In contrast to adults with SSD, a trial with re-routing technologies is not recommended for children with SSD since they may not be mature enough to appropriately control the device in specific environments (e.g., poorer signal is on the side of the affected ear and being routed over to the better ear). Also, the task force recommended counseling families about the influence of SSD on auditory development, including difficulty with sound source localization and speech recognition, and about the value of consistent daily CI use and aural rehabilitation.
10. How do you evaluate outcomes for these patients?
There are two considerations when selecting how to evaluate benefit for CI users with SSD: how to isolate signals to the implanted ear, and why the patient/family pursued cochlear implantation.
One aim is to measure speech recognition with the CI alone in order to evaluate progress with the device over time and to determine whether the device is functioning appropriately (since declines in speech recognition are red flags for potential issues with the coding of the signal or functioning of the internal device). This means we must isolate the signal from the contralateral, normal-hearing ear. Test methods may include presenting the recorded stimulus via direct audio input (DAI) or in the sound field with the contralateral ear either plugged or masked and a circumaural headphone placed over the pinna. Emily Buss and colleagues (2018) found similar CNC word recognition for adults when tested via DAI versus in the sound field with masking presented via an insert phone and the circumaural headphone placed over the pinna. Importantly, children may be more susceptible to masking, thus the task force recommended DAI as the test method for assessing performance with the CI alone for pediatric CI users with SSD.
When deciding how to assess outcomes with the CI, we also consider the primary reasons why the patient/family pursued cochlear implantation. The majority of patients with SSD report that they are pursuing cochlear implantation due to difficulty understanding speech in noise and with sound localization (Deep et al., 2021). Considering this, the task forces recommended the addition of spatial hearing abilities to the test battery in order to evaluate outcomes when listening with the CI plus the normal-hearing ear. A two-loudspeaker set-up allows for the assessment of speech recognition in noise; clinical protocols are not yet available for this loudspeaker set-up to assess localization. With a two-loudspeaker set-up, the audiologist can assess the effects of binaural summation, acoustic head-shadow, and binaural squelch by presenting the target from the front loudspeaker (0° azimuth) and the masker from the same loudspeaker (co-located), the loudspeaker towards the normal-hearing ear, or the loudspeaker towards the CI-ear, respectively. Performance in the best-aided condition (CI+NH) can be compared to preoperative abilities or when the patient is listening with the normal-hearing ear alone to assess the benefit of CI use.
There are also subjective questionnaires that can be administered to assess perceived spatial hearing abilities with the CI as compared to preoperative perceptions, such as the Speech, Spatial, and Qualities of Hearing Scale (Gatehouse & Noble, 2004). Responses to subjective questionnaires can be used to better understand the perceived difficulties of the patient at the preoperative evaluation and the perceived benefits of CI use over time that may not be captured with traditional speech recognition assessments (e.g., perceived listening effort; Lopez et al., 2021).
11. Is there anything else we should think about when evaluating performance for these patients?
Yes, the angular separation between the loudspeakers should be considered when evaluating the spatial hearing over time for a CI user. This is particularly important when evaluating a CI user who has transferred to your clinic and has performance score records, or if you work at a clinic with multiple soundbooths with different loudspeaker separations.
Kelly Anderson and colleagues (2022) compared speech recognition in noise scores when the two loudspeakers (one presenting the target speech and the other presenting the masking noise) were separated by 45° or by 90°. They found that CI users with SSD had significantly better performance when the loudspeakers were separated by 90° - which showed that listeners benefit from the larger separation between the target speech and the masking noise as compared to the 45° separation.
The takeaway here is to ensure that the test set-up is the same when comparing a patient’s speech recognition in spatially-separated noise between visits.
12. Does recommended aural habilitation/rehabilitation differ for CI users with SSD as compared to traditional CI candidates with bilateral hearing loss?
Aural habilitation/rehabilitation is recommended for children and adult CI users with SSD (Dillon, Kocharyan, et al., 2022; Park et al., 2022), though research is needed on the optimal frequency of auditory training and the most effective procedures. Methods for auditory training have included presenting the stimuli directly to the CI via DAI and training in a bilateral listening condition to support spatial hearing abilities (Dillon, Buss, Anderson, et al., 2017; Nawaz, McNeill, & Greenberg, 2014; Távora-Vieira & Marino, 2019; Van de Heyning et al., 2016)
13. This is all great information. What other new developments in the cochlear implant field do I need to catch up on?
A question underlying many research studies in the field is what factors contribute to the variability in individual CI and EAS user outcomes. One factor under active investigation is electric frequency-to-place mismatch, which is the discrepancy between the frequency information presented by an electrode contact and the cochlear place frequencies. Electric frequency-to-place mismatches occur because the default mapping procedures for CI and EAS devices do not account for the placement of the electrode array for an individual patient. Electrode arrays differ in length and design (e.g., lateral wall versus perimodiolar). When combining the variability in electrode array length and design with the variability in cochlear anatomy and surgical approach, it is not surprising that we see wide variability in the angular insertion depth (AID) across CI recipients – even for recipients of the same electrode array (Canfarotta et al., 2020; Landsberger et al., 2015).
We talked about how the default mapping procedures for CI and EAS devices aim to provide the patient with the full speech spectrum by distributing the frequency information across the active electrode channels. The distribution of frequency information with the default mapping procedures generally follows the tonotopic organization of the cochlea by distributing low to high frequency information across the electrode array. This procedure does not account for the actual placement of the electrode array relative to the cochlear place frequency – thus, the majority of CI patients are mapped with electric frequency-to-place mismatches (Canfarotta et al., 2020).
14. How can you figure out the actual placement of a patient’s electrode array?
Researchers and clinicians have been using imaging such as CT scans or x-ray to evaluate the placement of the electrode array (Canfarotta et al., 2019; Giardina et al., 2020; Noble et al., 2012; Skinner et al., 2002; Teymouri et al., 2011; Zhao et al., 2019). For the purposes of calculating electric frequency-to-place mismatch, imaging can be used to estimate the AID of each electrode contact. Electrode contacts placed more apically will have a deeper/higher AID, and electrode contacts placed more basally will have a shallower/lower AID. When you know the AID of a specific electrode contact, then you can plug that value into a frequency-to-place function to calculate the cochlear place frequency. Electric frequency-to-place mismatches are determined by comparing the frequency information being presented by the electrode contact with the cochlear place frequency.
15. I think I’m following you, but just barely—the notion is that we may want maps that match place frequencies?
Yes. There is longstanding evidence from simulation studies and with CI users that maps with electric frequency-to-place mismatches negatively influence speech recognition as compared to maps that match the frequency information with the cochlear place frequencies, which we refer to as place-based maps (Başkent & Shannon, 2003, 2007; Fu, Shannon, & Galvin, 2002; Shannon, Zeng, & Wygonski, 1998). Some CI users may acclimate to electric frequency-to-place mismatches and improve on measures of pitch perception or speech recognition with auditory training or long-term device use (Fu & Galvin, 2003; Fu et al., 2002; Li & Fu, 2007; Li, Galvin, & Fu, 2009; Reiss et al., 2007, 2014; Rosen, Faulkner, & Wilkinson, 1999; Smalt et al., 2013; Svirsky et al., 2015; Vermeire et al., 2015). However, not all CI users fully acclimate to electric frequency-to-place mismatches (Reiss et al., 2007, 2014; Sagi et al., 2010; Svirsky et al., 2015), and there are likely individual differences in the ability to acclimate to electric frequency-to-place mismatches (Smith & Winn, 2021).
In the case of EAS, simulation data and early data from EAS users suggest that electric frequency-to-place mismatches also negatively impact speech recognition and that better outcomes may be achieved with place-based maps (Dillon, Canfarotta, et al., 2021, 2023; Dillon, O’Connell, et al., 2022; Fu, Galvin, & Wang, 2017; Willis et al., 2020). The ability of EAS users to acclimate to electric frequency-to-place mismatches with long-term device use remains unknown.
Electric frequency-to-place mismatches also create a discrepancy with the frequency information from the contralateral ear, resulting in interaural mismatches. We have learned from simulations and studies of bilateral CI users that interaural mismatches negatively influence the ability to benefit from binaural cues (Goupell et al., 2018, 2013; Kan et al., 2013). More recently, this same effect has been observed in simulations of CI with SSD (Wess, Brungart, & Bernstein, 2017). Taken together, we think that CI and EAS users may benefit with maps with no or minimal electric frequency-to-place mismatches.
16. How can you minimize these electric frequency-to-place mismatches?
One way to minimize electric frequency-to-place mismatches is to implant a long electrode array with electrode contacts that end up near the cochlear place frequencies of a default map. In a prospective, randomized study investigating the effects of electrode array length on speech recognition, CI users implanted with a 31.5-mm electrode array experienced better speech recognition than CI users implanted with a 24-mm electrode array (Buchman et al., 2014). This pattern of performance was observed out to 12 months post-activation. In a follow-up report, the benefit of a longer electrode array was observed to continue through 4 years of CI use (Canfarotta et al., 2021).
A takeaway from those studies is that implanting a longer electrode array minimizes potential electric frequency-to-place mismatches when using the default mapping procedure. However, longer electrode arrays may not always be the preferred choice – like in the case of a patient with normal low-to-mid frequency hearing.
17. What about the patients that have already undergone cochlear implantation?
We are currently investigating the effectiveness of a place-based mapping procedure that eliminates electric-frequency-to-place mismatches for CI and EAS users. With our place-based mapping procedure, we use imaging to identify the AID of each electrode contact, calculate the cochlear place frequencies, and then adjust the electric filter frequencies for electrode contacts in the low- and mid-frequency region to match the cochlear place frequencies. The remaining high-frequency information is logarithmically distributed across the basal contacts. We decided to take this approach as opposed to deactivating electrode contacts that are above the upper filter frequency (i.e., 8500 Hz) since the spectral detail of speech tends to be more informative at low and mid frequencies than at high frequencies. We think that individualizing the map settings for each patient may help them hear their best faster because they are not having to acclimate to electric frequency-to-place mismatches.
We have also been piloting whether there is a preferred frequency-to-place function for our place-based mapping procedure. In one study, we found that average speech recognition measured acutely after activation of the CI or EAS device was better with a spiral ganglion frequency-to-place function as compared to an organ of Corti function (Dillon, Canfarotta, Buss, & O’Connell, 2021). More recently, we have been collaborating with the team from Western University using their Synchrotron Radiation-Artificial Intelligence (SR-AI) function, that incorporates more information about the patient’s cochlear anatomy than previous functions. The early data demonstrate that some patients experience better early performance with place-based maps using the SR-AI function as compared to alternative functions (Dillon, Helpard, et al., 2023).
Also, there are tools now available for clinical use that conduct similar procedures automatically. For example, MED-EL Corporation has the OTOPLAN software that calculates AID and estimates cochlear place frequencies, which can be uploaded to their clinical software for their mapping procedure aimed at eliminating electric frequency-to-place mismatches.
18. You mentioned the differences between mapping a CI-alone as compared to an EAS device. Are there additional differences when using this place-based mapping procedure?
Yes, I am glad you asked that! For EAS users, we are also thinking about where the electrode contacts are relative to the functional acoustic hearing region (e.g., ≤ 80 dB HL). Electric stimulation from apical electrode contacts can mask the acoustic signal, an effect described as electric-on-acoustic interference (Imsiecke et al., 2020; Kipping, Krüger, & Nogueira, 2020; Krüger, Büchner, & Nogueira, 2017; Lin et al., 2011; Stronks et al., 2010, 2012). Electric-on-acoustic interference can limit the ability to benefit from the low-frequency acoustic information, resulting in poorer speech recognition.
Our place-based mapping procedure limits electric-on-acoustic interference by identifying the electrode contacts within the functional acoustic hearing region via imaging and turning the stimulation levels for those channels below the patient’s detection threshold.
For patients that lose their hearing post-activation, one benefit to place-based mapping, as compared to default mapping, is that the patient maintains the same electric filter frequencies. With the default mapping procedure, if the acoustic hearing changes, then the acoustic cutoff frequency changes, and the electric filter frequencies change. This requires the patient to acclimate to new filter frequency assignments. With the place-based mapping procedure, acoustic settings are adjusted to prescriptive targets using the unaided hearing thresholds and the electric filter frequencies stay the same. For patients with electrode contacts that had stimulation levels lowered, the stimulation levels can be raised to their maximum comfortable loudness to electrically represent the associated speech information.
19. Is all this work to personalize the mapping of the EAS device worth the effort?
We think it is! Data from EAS simulation studies show that listeners have significantly poorer speech recognition with default maps having electric frequency-to-place mismatches than with place-based maps – even when there is a gap between the frequency information represented acoustically versus electrically (Dillon, Canfarotta, Buss, Hopfinger, et al., 2021; Fu et al., 2017; Willis et al., 2020). We have been conducting a prospective study of EAS users who are randomized at device activation to listen with a default or place-based map and listen exclusively to the assigned map for the first year of device use. Our preliminary data at 6 months post-activation show that EAS users with larger magnitudes of electric frequency-to-place mismatches have poorer monaural speech recognition as compared to those with minimal electric mismatches (Dillon, Canfarotta, et al., 2023). We are continuing to investigate the long-term results with default versus place-based maps as well as performance on tasks for binaural hearing (e.g., sound source localization and speech recognition in noise).
We are also looking into whether place-based maps would benefit CI and EAS users with long-term listening experience with default maps. There is evidence that CI patients can continue to acclimate to changes in their electric filter frequencies even after long-term CI use (Reiss et al., 2014). We have been investigating whether experienced CI and EAS users with various magnitudes of electric frequency-to-place mismatches with their default maps benefit on measures of monaural and binaural hearing if switched to place-based maps.
20. Thanks for all the updates. As I expected, there’s always a lot of advances in the area of cochlear implants. It must be an exciting area for you to work?
It definitely is. Working with a team of audiologists, surgeons, speech-language pathologists, and researchers on projects aimed at understanding how we can support the best outcomes for children and adults with hearing loss is a dream come true.
References
Adunka, O. F., Dillon, M. T., Adunka, M. C., King, E. R., Pillsbury, H. C., & Buchman, C. A. (2013). Hearing preservation and speech perception outcomes with electric-acoustic stimulation after 12 months of listening experience. The Laryngoscope, 123(10), 2509–2515. https://doi.org/10.1002/lary.23741
Anderson, K. M., Buss, E., Rooth, M. A., Richter, M. E., Overton, A. B., Brown, K. D., & Dillon, M. T. (2022). Masked Speech Recognition as a Function of Masker Location for Cochlear Implant Users With Single-Sided Deafness. American Journal of Audiology, 31(3), 757–763. https://doi.org/10.1044/2022_AJA-21-00268
Arndt, S., Aschendorff, A., Laszig, R., Beck, R., Schild, C., Kroeger, S., … Wesarg, T. (2011). Comparison of pseudobinaural hearing to real binaural hearing rehabilitation after cochlear implantation in patients with unilateral deafness and tinnitus. Otology & Neurotology, 32(1), 39–47. https://doi.org/10.1097/MAO.0b013e3181fcf271
Arndt, S., Prosse, S., Laszig, R., Wesarg, T., Aschendorff, A., & Hassepass, F. (2015). Cochlear implantation in children with single-sided deafness: does aetiology and duration of deafness matter? Audiology & Neuro-Otology, 20 Suppl 1(1), 21–30. https://doi.org/10.1159/000380744
Baskent, D., & Shannon, R. V. (2003). Speech recognition under conditions of frequency-place compression and expansion. The Journal of the Acoustical Society of America, 113(4 Pt 1), 2064–2076. https://doi.org/10.1121/1.1558357
Başkent, D., & Shannon, R. V. (2007). Combined effects of frequency compression-expansion and shift on speech recognition. Ear and Hearing, 28(3), 277–289. https://doi.org/10.1097/AUD.0b013e318050d398
Beck, R. L., Aschendorff, A., Hassepaß, F., Wesarg, T., Kröger, S., Jakob, T. F., & Arndt, S. (2017). Cochlear implantation in children with congenital unilateral deafness: A case series. Otology & Neurotology, 38(10), e570–e576. https://doi.org/10.1097/MAO.0000000000001597
Benchetrit, L., Ronner, E. A., Anne, S., & Cohen, M. S. (2021). Cochlear Implantation in Children With Single-Sided Deafness: A Systematic Review and Meta-analysis. JAMA Otolaryngology-- Head & Neck Surgery, 147(1), 58–69. https://doi.org/10.1001/jamaoto.2020.3852
Brown, K. D., Dillon, M. T., & Park, L. R. (2022). Benefits of cochlear implantation in childhood unilateral hearing loss (CUHL trial). The Laryngoscope, 132 Suppl 6(Suppl 6), S1–S18. https://doi.org/10.1002/lary.29853
Buchman, C. A., Dillon, M. T., King, E. R., Adunka, M. C., Adunka, O. F., & Pillsbury, H. C. (2014). Influence of cochlear implant insertion depth on performance: a prospective randomized trial. Otology & Neurotology, 35(10), 1773–1779. https://doi.org/10.1097/MAO.0000000000000541
Büchner, A., Illg, A., Majdani, O., & Lenarz, T. (2017). Investigation of the effect of cochlear implant electrode length on speech comprehension in quiet and noise compared with the results with users of electro-acoustic-stimulation, a retrospective analysis. Plos One, 12(5), e0174900. https://doi.org/10.1371/journal.pone.0174900
Buss, E., Dillon, M. T., Rooth, M. A., King, E. R., Deres, E. J., Buchman, C. A., … Brown, K. D. (2018). Effects of cochlear implantation on binaural hearing in adults with unilateral hearing loss. Trends in Hearing, 22, 2331216518771173. https://doi.org/10.1177/2331216518771173
Canfarotta, M. W., Dillon, M. T., Buchman, C. A., Buss, E., O’Connell, B. P., Rooth, M. A., … Brown, K. D. (2021). Long-Term Influence of Electrode Array Length on Speech Recognition in Cochlear Implant Users. The Laryngoscope, 131(4), 892–897. https://doi.org/10.1002/lary.28949
Canfarotta, M. W., Dillon, M. T., Buss, E., Pillsbury, H. C., Brown, K. D., & O’Connell, B. P. (2019). Validating a New Tablet-based Tool in the Determination of Cochlear Implant Angular Insertion Depth. Otology & Neurotology, 40(8), 1006–1010. https://doi.org/10.1097/MAO.0000000000002296
Canfarotta, M. W., Dillon, M. T., Buss, E., Pillsbury, H. C., Brown, K. D., & O’Connell, B. P. (2020). Frequency-to-Place Mismatch: Characterizing Variability and the Influence on Speech Perception Outcomes in Cochlear Implant Recipients. Ear and Hearing, 41(5), 1349–1361. https://doi.org/10.1097/AUD.0000000000000864
Deep, N. L., Spitzer, E. R., Shapiro, W. H., Waltzman, S. B., Roland, J. T., & Friedmann, D. R. (2021). Cochlear Implantation in Adults With Single-sided Deafness: Outcomes and Device Use. Otology & Neurotology, 42(3), 414–423. https://doi.org/10.1097/MAO.0000000000002955
Dillon, M. T., Buss, E., Anderson, M. L., King, E. R., Deres, E. J., Buchman, C. A., … Pillsbury, H. C. (2017). Cochlear implantation in cases of unilateral hearing loss: initial localization abilities. Ear and Hearing, 38(5), 611–619. https://doi.org/10.1097/AUD.0000000000000430
Dillon, M. T., Buss, E., O’Connell, B. P., Rooth, M. A., King, E. R., Bucker, A. L., … Brown, K. D. (2020). Low-Frequency Hearing Preservation With Long Electrode Arrays: Inclusion of Unaided Hearing Threshold Assessment in the Postoperative Test Battery. American Journal of Audiology, 29(1), 1–5. https://doi.org/10.1044/2019_AJA-19-00045
Dillon, M. T., Buss, E., Pillsbury, H. C., Adunka, O. F., Buchman, C. A., & Adunka, M. C. (2014). Effects of hearing aid settings for electric-acoustic stimulation. Journal of the American Academy of Audiology, 25(2), 133–140. https://doi.org/10.3766/jaaa.25.2.2
Dillon, M. T., Buss, E., Rooth, M. A., King, E. R., Deres, E. J., Buchman, C. A., … Brown, K. D. (2017). Effect of Cochlear Implantation on Quality of Life in Adults with Unilateral Hearing Loss. Audiology & Neuro-Otology, 22(4–5), 259–271. https://doi.org/10.1159/000484079
Dillon, M. T., Canfarotta, M. W., Buss, E., Hopfinger, J., & O’Connell, B. P. (2021). Effectiveness of Place-based Mapping in Electric-Acoustic Stimulation Devices. Otology & Neurotology, 42(1), 197–202. https://doi.org/10.1097/MAO.0000000000002965
Dillon, M. T., Canfarotta, M. W., Buss, E., & O’Connell, B. P. (2021). Comparison of Speech Recognition With an Organ of Corti Versus Spiral Ganglion Frequency-to-Place Function in Place-Based Mapping of Cochlear Implant and Electric-Acoustic Stimulation Devices. Otology & Neurotology, 42(5), 721–725. https://doi.org/10.1097/MAO.0000000000003070
Dillon, M. T., Canfarotta, M. W., Buss, E., Rooth, M. A., Richter, M. E., Overton, A. B., … O’Connell, B. P. (2023). Influence of Electric Frequency-to-Place Mismatches on the Early Speech Recognition Outcomes for Electric-Acoustic Stimulation Users. American Journal of Audiology, 1–10. https://doi.org/10.1044/2022_AJA-21-00254
Dillon, M. T., Helpard, L., Brown, K. D., Selleck, A. M., Richter, M. E., Rooth, M. A., … Agrawal, S. (2023). Influence of the Frequency-to-Place Function on Recognition with Place-Based Cochlear Implant Maps. The Laryngoscope. https://doi.org/10.1002/lary.30710
Dillon, M. T., Kocharyan, A., Daher, G. S., Carlson, M. L., Shapiro, W. H., Snapp, H. A., & Firszt, J. B. (2022). American Cochlear Implant Alliance Task Force Guidelines for Clinical Assessment and Management of Adult Cochlear Implantation for Single-Sided Deafness. Ear and Hearing. https://doi.org/10.1097/AUD.0000000000001260
Dillon, M. T., O’Connell, B. P., Canfarotta, M. W., Buss, E., & Hopfinger, J. (2022). Effect of Place-Based Versus Default Mapping Procedures on Masked Speech Recognition: Simulations of Cochlear Implant Alone and Electric-Acoustic Stimulation. American Journal of Audiology, 31(2), 322–337. https://doi.org/10.1044/2022_AJA-21-00123
Dillon, M. T., Rooth, M. A., Canfarotta, M. W., Richter, M. E., Thompson, N. J., & Brown, K. D. (2022). Sound Source Localization by Cochlear Implant Recipients with Normal Hearing in the Contralateral Ear: Effects of Spectral Content and Duration of Listening Experience. Audiology & Neuro-Otology, 1–12. https://doi.org/10.1159/000523969
Dunn, C. C., Perreau, A., Gantz, B., & Tyler, R. S. (2010). Benefits of localization and speech perception with multiple noise sources in listeners with a short-electrode cochlear implant. Journal of the American Academy of Audiology, 21(1), 44–51.
Ehrmann-Mueller, D., Kurz, A., Kuehn, H., Rak, K., Mlynski, R., Hagen, R., & Shehata-Dieler, W. (2020). Usefulness of cochlear implantation in children with single sided deafness. International Journal of Pediatric Otorhinolaryngology, 130, 109808. https://doi.org/10.1016/j.ijporl.2019.109808
Firszt, J. B., Holden, L. K., Reeder, R. M., Waltzman, S. B., & Arndt, S. (2012). Auditory abilities after cochlear implantation in adults with unilateral deafness: a pilot study. Otology & Neurotology, 33(8), 1339–1346. https://doi.org/10.1097/MAO.0b013e318268d52d
Fu, Q.-J., & Galvin, J. J. (2003). The effects of short-term training for spectrally mismatched noise-band speech. The Journal of the Acoustical Society of America, 113(2), 1065–1072. https://doi.org/10.1121/1.1537708
Fu, Q.-J., Galvin, J. J., & Wang, X. (2017). Integration of acoustic and electric hearing is better in the same ear than across ears. Scientific Reports, 7(1), 12500. https://doi.org/10.1038/s41598-017-12298-3
Fu, Q.-J., Shannon, R. V., & Galvin, J. J. (2002). Perceptual learning following changes in the frequency-to-electrode assignment with the Nucleus-22 cochlear implant. The Journal of the Acoustical Society of America, 112(4), 1664–1674. https://doi.org/10.1121/1.1502901
Galvin, J. J., Fu, Q.-J., Wilkinson, E. P., Mills, D., Hagan, S. C., Lupo, J. E., … Shannon, R. V. (2019). Benefits of Cochlear Implantation for Single-Sided Deafness: Data From the House Clinic-University of Southern California-University of California, Los Angeles Clinical Trial. Ear and Hearing, 40(4), 766–781. https://doi.org/10.1097/AUD.0000000000000671
Gantz, B. J., Dunn, C., Oleson, J., Hansen, M., Parkinson, A., & Turner, C. (2016). Multicenter clinical trial of the Nucleus Hybrid S8 cochlear implant: Final outcomes. The Laryngoscope, 126(4), 962–973. https://doi.org/10.1002/lary.25572
Gatehouse, S., & Noble, W. (2004). The speech, spatial and qualities of hearing scale (SSQ). International Journal of Audiology, 43(2), 85–99. https://doi.org/10.1080/14992020400050014
Gfeller, K. E., Olszewski, C., Turner, C., Gantz, B., & Oleson, J. (2006). Music perception with cochlear implants and residual hearing. Audiology & Neuro-Otology, 11 Suppl 1, 12–15. https://doi.org/10.1159/000095608
Giardina, C. K., Canfarotta, M. W., Thompson, N. J., Fitzpatrick, D. C., Hodge, S. E., Baker, J., & O’Connell, B. P. (2020). Assessing Cochlear Implant Insertion Angle From an Intraoperative X-ray Using a Rotating 3D Helical Scala Tympani Model. Otology & Neurotology, 41(6), e686–e694. https://doi.org/10.1097/MAO.0000000000002638
Gifford, R. H., Dorman, M. F., Skarzynski, H., Lorens, A., Polak, M., Driscoll, C. L. W., … Buchman, C. A. (2013). Cochlear implantation with hearing preservation yields significant benefit for speech recognition in complex listening environments. Ear and Hearing, 34(4), 413–425. https://doi.org/10.1097/AUD.0b013e31827e8163
Gifford, R. H., Grantham, D. W., Sheffield, S. W., Davis, T. J., Dwyer, R., & Dorman, M. F. (2014). Localization and interaural time difference (ITD) thresholds for cochlear implant recipients with preserved acoustic hearing in the implanted ear. Hearing Research, 312, 28–37. https://doi.org/10.1016/j.heares.2014.02.007
Goupell, M. J., Stoelb, C. A., Kan, A., & Litovsky, R. Y. (2018). The effect of simulated interaural frequency mismatch on speech understanding and spatial release from masking. Ear and Hearing, 39(5), 895–905. https://doi.org/10.1097/AUD.0000000000000541
Goupell, M. J., Stoelb, C., Kan, A., & Litovsky, R. Y. (2013). Effect of mismatched place-of-stimulation on the salience of binaural cues in conditions that simulate bilateral cochlear-implant listening. The Journal of the Acoustical Society of America, 133(4), 2272–2287. https://doi.org/10.1121/1.4792936
Gstoettner, W. K., van de Heyning, P., O’Connor, A. F., Morera, C., Sainz, M., Vermeire, K., … Adunka, O. F. (2008). Electric acoustic stimulation of the auditory system: results of a multi-centre investigation. Acta Oto-Laryngologica, 128(9), 968–975. https://doi.org/10.1080/00016480701805471
Helbig, S., Baumann, U., Hey, C., & Helbig, M. (2011). Hearing preservation after complete cochlear coverage in cochlear implantation with the free-fitting FLEXSOFT electrode carrier. Otology & Neurotology, 32(6), 973–979. https://doi.org/10.1097/MAO.0b013e31822558c4
Helbig, S., Rajan, G. P., Stöver, T., Lockley, M., Kuthubutheen, J., & Green, K. M. (2013). Hearing preservation after cochlear reimplantation. Otology & Neurotology, 34(1), 61–65. https://doi.org/10.1097/MAO.0b013e318277a44e
Helbig, S., Van de Heyning, P., Kiefer, J., Baumann, U., Kleine-Punte, A., Brockmeier, H., … Gstoettner, W. (2011). Combined electric acoustic stimulation with the PULSARCI(100) implant system using the FLEX(EAS) electrode array. Acta Oto-Laryngologica, 131(6), 585–595. https://doi.org/10.3109/00016489.2010.544327
Hollis, E. S., Canfarotta, M. W., Dillon, M. T., Rooth, M. A., Bucker, A. L., Dillon, S. A., … Brown, K. D. (2021). Initial Hearing Preservation Is Correlated With Cochlear Duct Length in Fully-inserted Long Flexible Lateral Wall Arrays. Otology & Neurotology. https://doi.org/10.1097/MAO.0000000000003181
Imsiecke, M., Büchner, A., Lenarz, T., & Nogueira, W. (2020). Psychoacoustic and electrophysiological electric-acoustic interaction effects in cochlear implant users with ipsilateral residual hearing. Hearing Research, 386, 107873. https://doi.org/10.1016/j.heares.2019.107873
Jensen, M. J., Isaac, H., Hernandez, H., Oleson, J., Dunn, C., Gantz, B. J., & Hansen, M. R. (2022). Timing of acoustic hearing changes after cochlear implantation. The Laryngoscope, 132(10), 2036–2043. https://doi.org/10.1002/lary.29984
Kan, A., Stoelb, C., Litovsky, R. Y., & Goupell, M. J. (2013). Effect of mismatched place-of-stimulation on binaural fusion and lateralization in bilateral cochlear-implant users. The Journal of the Acoustical Society of America, 134(4), 2923–2936. https://doi.org/10.1121/1.4820889
Kipping, D., Krüger, B., & Nogueira, W. (2020). The role of electroneural versus electrophonic stimulation on psychoacoustic electric-acoustic masking in cochlear implant users with residual hearing. Hearing Research, 395, 108036. https://doi.org/10.1016/j.heares.2020.108036
Krüger, B., Büchner, A., & Nogueira, W. (2017). Simultaneous masking between electric and acoustic stimulation in cochlear implant users with residual low-frequency hearing. Hearing Research, 353, 185–196. https://doi.org/10.1016/j.heares.2017.06.014
Landsberger, D. M., Svrakic, M., Roland, J. T., & Svirsky, M. (2015). The relationship between insertion angles, default frequency allocations, and spiral ganglion place pitch in cochlear implants. Ear and Hearing, 36(5), e207-13. https://doi.org/10.1097/AUD.0000000000000163
Li, T., & Fu, Q.-J. (2007). Perceptual adaptation to spectrally shifted vowels: training with nonlexical labels. Journal of the Association for Research in Otolaryngology, 8(1), 32–41. https://doi.org/10.1007/s10162-006-0059-2
Li, T., Galvin, J. J., & Fu, Q.-J. (2009). Interactions between unsupervised learning and the degree of spectral mismatch on short-term perceptual adaptation to spectrally shifted speech. Ear and Hearing, 30(2), 238–249. https://doi.org/10.1097/AUD.0b013e31819769ac
Lin, P., Turner, C. W., Gantz, B. J., Djalilian, H. R., & Zeng, F.-G. (2011). Ipsilateral masking between acoustic and electric stimulations. The Journal of the Acoustical Society of America, 130(2), 858–865. https://doi.org/10.1121/1.3605294
Lopez, E. M., Dillon, M. T., Park, L. R., Rooth, M. A., Richter, M. E., Thompson, N. J., … Brown, K. D. (2021). Influence of cochlear implant use on perceived listening effort in adult and pediatric cases of unilateral and asymmetric hearing loss. Otology & Neurotology, 42(9), e1234–e1241. https://doi.org/10.1097/MAO.0000000000003261
Marinelli, J. P., Sydlowski, S. A., & Carlson, M. L. (2022). Cochlear implant awareness in the united states: A national survey of 15,138 adults. Seminars in Hearing, 43(4), 317–323. https://doi.org/10.1055/s-0042-1758376
Mick, P., Amoodi, H., Shipp, D., Friesen, L., Symons, S., Lin, V., … Chen, J. (2014). Hearing preservation with full insertion of the FLEXsoft electrode. Otology & Neurotology, 35(1), e40-4. https://doi.org/10.1097/MAO.0b013e318291c66d
Nassiri, A. M., Sorkin, D. L., & Carlson, M. L. (2022). Current estimates of cochlear implant utilization in the united states. Otology & Neurotology, 43(5), e558–e562. https://doi.org/10.1097/MAO.0000000000003513
Nawaz, S., McNeill, C., & Greenberg, S. L. (2014). Improving sound localization after cochlear implantation and auditory training for the management of single-sided deafness. Otology & Neurotology, 35(2), 271–276. https://doi.org/10.1097/MAO.0000000000000257
Noble, J. H., Gifford, R. H., Labadie, R. F., & Dawant, B. M. (2012). Statistical shape model segmentation and frequency mapping of cochlear implant stimulation targets in CT. Medical Image Computing and Computer-Assisted Intervention, 15(Pt 2), 421–428. https://doi.org/10.1007/978-3-642-33418-4_52
Park, L. R., Dillon, M. T., Buss, E., & Brown, K. D. (2023). Two-Year Outcomes of Cochlear Implant Use for Children With Unilateral Hearing Loss: Benefits and Comparison to Children With Normal Hearing. Ear and Hearing. https://doi.org/10.1097/AUD.0000000000001353
Park, L. R., Griffin, A. M., Sladen, D. P., Neumann, S., & Young, N. M. (2022). American Cochlear Implant Alliance Task Force Guidelines for Clinical Assessment and Management of Cochlear Implantation in Children With Single-Sided Deafness. Ear and Hearing, 43(2), 255–267. https://doi.org/10.1097/AUD.0000000000001204
Park, L. R., Preston, E., Eskridge, H., King, E. R., & Brown, K. D. (2021). Sound opportunities: factors that impact referral for pediatric cochlear implant evaluation. The Laryngoscope, 131(12), E2904–E2910. https://doi.org/10.1002/lary.29686
Pillsbury, H. C., Dillon, M. T., Buchman, C. A., Staecker, H., Prentiss, S. M., Ruckenstein, M. J., … Adunka, O. F. (2018). Multicenter US Clinical Trial With an Electric-Acoustic Stimulation (EAS) System in Adults: Final Outcomes. Otology & Neurotology, 39(3), 299–305. https://doi.org/10.1097/MAO.0000000000001691
Ramos Macías, Á., Borkoski-Barreiro, S. A., Falcón González, J. C., de Miguel Martínez, I., & Ramos de Miguel, Á. (2019). Single-sided deafness and cochlear implantation in congenital and acquired hearing loss in children. Clinical Otolaryngology, 44(2), 138–143. https://doi.org/10.1111/coa.13245
Reiss, L A J, Turner, C. W., Karsten, S. A., & Gantz, B. J. (2014). Plasticity in human pitch perception induced by tonotopically mismatched electro-acoustic stimulation. Neuroscience, 256, 43–52. https://doi.org/10.1016/j.neuroscience.2013.10.024
Reiss, Lina A J, Turner, C. W., Erenberg, S. R., & Gantz, B. J. (2007). Changes in pitch with a cochlear implant over time. Journal of the Association for Research in Otolaryngology, 8(2), 241–257. https://doi.org/10.1007/s10162-007-0077-8
Rosen, S., Faulkner, A., & Wilkinson, L. (1999). Adaptation by normal listeners to upward spectral shifts of speech: implications for cochlear implants. The Journal of the Acoustical Society of America, 106(6), 3629–3636.
Sagi, E., Fu, Q.-J., Galvin, J. J., & Svirsky, M. A. (2010). A model of incomplete adaptation to a severely shifted frequency-to-electrode mapping by cochlear implant users. Journal of the Association for Research in Otolaryngology, 11(1), 69–78. https://doi.org/10.1007/s10162-009-0187-6
Shannon, R. V., Zeng, F. G., & Wygonski, J. (1998). Speech recognition with altered spectral distribution of envelope cues. The Journal of the Acoustical Society of America, 104(4), 2467–2476.
Sheffield, S. W., & Gifford, R. H. (2014). The benefits of bimodal hearing: effect of frequency region and acoustic bandwidth. Audiology & Neuro-Otology, 19(3), 151–163. https://doi.org/10.1159/000357588
Skinner, M. W., Ketten, D. R., Holden, L. K., Harding, G. W., Smith, P. G., Gates, G. A., … Blocker, B. (2002). CT-derived estimation of cochlear morphology and electrode array position in relation to word recognition in Nucleus-22 recipients. Journal of the Association for Research in Otolaryngology, 3(3), 332–350. https://doi.org/10.1007/s101620020013
Sladen, D. P., Frisch, C. D., Carlson, M. L., Driscoll, C. L. W., Torres, J. H., & Zeitler, D. M. (2017). Cochlear implantation for single-sided deafness: A multicenter study. The Laryngoscope, 127(1), 223–228. https://doi.org/10.1002/lary.26102
Smalt, C. J., Gonzalez-Castillo, J., Talavage, T. M., Pisoni, D. B., & Svirsky, M. A. (2013). Neural correlates of adaptation in freely-moving normal hearing subjects under cochlear implant acoustic simulations. Neuroimage, 82, 500–509. https://doi.org/10.1016/j.neuroimage.2013.06.001
Smith, M. L., & Winn, M. B. (2021). Individual variability in recalibrating to spectrally shifted speech: implications for cochlear implants. Ear and Hearing. https://doi.org/10.1097/AUD.0000000000001043
Sorkin, D. L. (2013). Cochlear implantation in the world’s largest medical device market: utilization and awareness of cochlear implants in the United States. Cochlear Implants International, 14 Suppl 1, S4-12. https://doi.org/10.1179/1467010013Z.000000000102
Stronks, H. C., Prijs, V. F., Chimona, T. S., Grolman, W., & Klis, S. F. L. (2012). Spatial overlap of combined electroacoustic stimulation determines the electrically evoked response in the guinea pig cochlea. Otology & Neurotology, 33(9), 1535–1542. https://doi.org/10.1097/MAO.0b013e318271c0b6
Stronks, H. C., Versnel, H., Prijs, V. F., & Klis, S. F. L. (2010). Suppression of the acoustically evoked auditory-nerve response by electrical stimulation in the cochlea of the guinea pig. Hearing Research, 259(1–2), 64–74. https://doi.org/10.1016/j.heares.2009.10.004
Suhling, M.-C., Majdani, O., Salcher, R., Leifholz, M., Büchner, A., Lesinski-Schiedat, A., & Lenarz, T. (2016). The impact of electrode array length on hearing preservation in cochlear implantation. Otology & Neurotology, 37(8), 1006–1015. https://doi.org/10.1097/MAO.0000000000001110
Svirsky, M. A., Talavage, T. M., Sinha, S., Neuburger, H., & Azadpour, M. (2015). Gradual adaptation to auditory frequency mismatch. Hearing Research, 322, 163–170. https://doi.org/10.1016/j.heares.2014.10.008
Sweeney, V., Dillon, M., Park, L., & Brown, K. D. (2022). Don’t defer, please refer! cochlear implantation candidacy as part of the hearing health care continuum. The Hearing Journal, 75(10), 8,9. https://doi.org/10.1097/01.HJ.0000891504.73227.12
Távora-Vieira, D., & Marino, R. (2019). Re-training the deaf ear: Auditory training for adult cochlear implant users with singlesided deafness. Cochlear Implants International, 20(5), 231–236. https://doi.org/10.1080/14670100.2019.1603652
Távora-Vieira, D., Marino, R., Acharya, A., & Rajan, G. P. (2015). The impact of cochlear implantation on speech understanding, subjective hearing performance, and tinnitus perception in patients with unilateral severe to profound hearing loss. Otology & Neurotology, 36(3), 430–436. https://doi.org/10.1097/MAO.0000000000000707
Távora-Vieira, D., & Rajan, G. P. (2015). Cochlear implantation in children with congenital and noncongenital unilateral deafness: a case series. Otology & Neurotology, 36(2), 235–239. https://doi.org/10.1097/MAO.0000000000000677
Teymouri, J., Hullar, T. E., Holden, T. A., & Chole, R. A. (2011). Verification of computed tomographic estimates of cochlear implant array position: a micro-CT and histologic analysis. Otology & Neurotology, 32(6), 980–986. https://doi.org/10.1097/MAO.0b013e3182255915
Thomas, J. P., Neumann, K., Dazert, S., & Voelter, C. (2017). Cochlear Implantation in Children With Congenital Single-Sided Deafness. Otology & Neurotology, 38(4), 496–503. https://doi.org/10.1097/MAO.0000000000001343
Thompson, N. J., Brown, K. D., Buss, E., Rooth, M. A., Richter, M. E., & Dillon, M. T. (2022). Long-Term Binaural Hearing Improvements for Cochlear Implant Users with Asymmetric Hearing Loss. The Laryngoscope. https://doi.org/10.1002/lary.30368
Thompson, N. J., Dillon, M. T., Bucker, A. L., King, E. R., Pillsbury, H. C., & Brown, K. D. (2019). Electric-Acoustic Stimulation After Reimplantation: Hearing Preservation and Speech Perception. i(2), e94–e98. https://doi.org/10.1097/MAO.0000000000002094
Thompson, N. J., Dillon, M. T., Buss, E., Rooth, M. A., Richter, M. E., Pillsbury, H. C., & Brown, K. D. (2022). Long-Term Improvement in Localization for Cochlear Implant Users with Single-Sided Deafness. The Laryngoscope. https://doi.org/10.1002/lary.30065
Thompson, N. J., Lopez, E. M., Dillon, M. T., Rooth, M. A., Richter, M. E., Pillsbury, H. C., & Brown, K. D. (2023). Cochlear Implantation for Unilateral and Asymmetric Hearing Loss: Long-Term Subjective Benefit. The Laryngoscope. https://doi.org/10.1002/lary.30608
Van de Heyning, P., Távora-Vieira, D., Mertens, G., Van Rompaey, V., Rajan, G. P., Müller, J., … Zernotti, M. E. (2016). Towards a Unified Testing Framework for Single-Sided Deafness Studies: A Consensus Paper. Audiology & Neuro-Otology, 21(6), 391–398. https://doi.org/10.1159/000455058
Van de Heyning, P., Vermeire, K., Diebl, M., Nopp, P., Anderson, I., & De Ridder, D. (2008). Incapacitating unilateral tinnitus in single-sided deafness treated by cochlear implantation. The Annals of Otology, Rhinology, and Laryngology, 117(9), 645–652. https://doi.org/10.1177/000348940811700903
Vermeire, K., Landsberger, D. M., Van de Heyning, P. H., Voormolen, M., Kleine Punte, A., Schatzer, R., & Zierhofer, C. (2015). Frequency-place map for electrical stimulation in cochlear implants: Change over time. Hearing Research, 326, 8–14. https://doi.org/10.1016/j.heares.2015.03.011
Vos, T. G., Park, L. R., Noxon, A. S., & Brown, K. D. (2022). Cochlear nerve deficiency in pediatric unilateral hearing loss and asymmetric hearing loss. Audiology & Neuro-Otology, 27(4), 328–335. https://doi.org/10.1159/000522566
Wanna, G. B., O’Connell, B. P., Francis, D. O., Gifford, R. H., Hunter, J. B., Holder, J. T., … Haynes, D. S. (2018). Predictive factors for short- and long-term hearing preservation in cochlear implantation with conventional-length electrodes. The Laryngoscope, 128(2), 482–489. https://doi.org/10.1002/lary.26714
Wess, J. M., Brungart, D. S., & Bernstein, J. G. W. (2017). The Effect of Interaural Mismatches on Contralateral Unmasking With Single-Sided Vocoders. Ear and Hearing, 38(3), 374–386. https://doi.org/10.1097/AUD.0000000000000374
Willis, S., Moore, B. C. J., Galvin, J. J., & Fu, Q.-J. (2020). Effects of noise on integration of acoustic and electric hearing within and across ears. Plos One, 15(10), e0240752. https://doi.org/10.1371/journal.pone.0240752
Zeitler, D. M., Sladen, D. P., DeJong, M. D., Torres, J. H., Dorman, M. F., & Carlson, M. L. (2019). Cochlear implantation for single-sided deafness in children and adolescents. International Journal of Pediatric Otorhinolaryngology, 118, 128–133. https://doi.org/10.1016/j.ijporl.2018.12.037
Zhang, T., Dorman, M. F., & Spahr, A. J. (2010). Information from the voice fundamental frequency (F0) region accounts for the majority of the benefit when acoustic stimulation is added to electric stimulation. Ear and Hearing, 31(1), 63–69. https://doi.org/10.1097/AUD.0b013e3181b7190c
Zhao, Y., Chakravorti, S., Labadie, R. F., Dawant, B. M., & Noble, J. H. (2019). Automatic graph-based method for localization of cochlear implant electrode arrays in clinical CT with sub-voxel accuracy. Medical Image Analysis, 52, 1–12. https://doi.org/10.1016/j.media.2018.11.005
Zwolan, T. A., Schvartz-Leyzac, K. C., & Pleasant, T. (2020). Development of a 60/60 guideline for referring adults for a traditional cochlear implant candidacy evaluation. Otology & Neurotology, 41(7), 895–900. https://doi.org/10.1097/MAO.0000000000002664
Citation
Dillon, M. (2023). 20Q: update on cochlear implants: hearing preservation, single-sided deafness, and personalized fitting. AudiologyOnline, Article 28633. Available at www.audiologyonline.com