From the Desk of Gus Mueller
The interest in tinnitus and the treatment of patients with tinnitus are topics that continue to expand each year. Your overwhelming response to our 20Q article last month on these topics supports this observation. We are back this month with more about tinnitus from our leading expert on the topic, Dr. Dick Salvi.
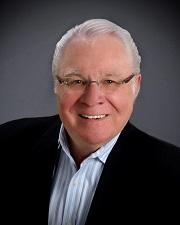
Richard Salvi, PhD, is Distinguished Professor in the Department of Communicative Disorders and Sciences at SUNY Buffalo. Dr. Salvi is co-founder—and continues to serve as director—of UB’s Center for Hearing and Deafness, one of the nation’s foremost hearing research groups.
Over the years, his research has involved the study of noise and ototoxic drug-induced hearing loss, inner ear physiology, central auditory plasticity and reorganization, hair cell regeneration and the use of stem cells to treat hearing loss.
In Dr. Salvi’s 20Q last month, he discussed the events that trigger or provoke tinnitus, the relationship between hearing loss and tinnitus, the neural generators of subjective tinnitus, and some interesting facts about somatic tinnitus.
This month, Dick will be touching on some of these same areas, with a focus on his work with animals, illustrating how this ongoing research helps us understand the origins and complexities of human tinnitus. Do animals actually have subjective tinnitus, you might ask? Seems possible that some might, and they certainly will have it when researchers induce tinnitus with drugs such as salicylate. These animal studies have helped expand our knowledge of the origins and neural correlates of both tinnitus and hyperacusis, including identification of some brain regions that might surprise you.
If you liked last month’s 20Q, you’ll find this one equally interesting. If by chance you missed last month, you might want to read that first. You’ll find both of them easy to read and digest, yet loaded with research-supported useful information.
Gus Mueller, PhDContributing Editor
March 2016
To browse the complete collection of 20Q with Gus Mueller CEU articles, please visit www.audiologyonline.com/20Q
20Q: Tinnitus and Hyperacusis - Beyond the Ear
Learning Objectives
- Participants will be able to explain how animal models are used in the study of the pathophysiology of tinnitus and hyperacusis.
- Participants will be able to describe findings from studies using animal models that investigated the origin and pathophysiology of tinnitus and hyperacusis.
- Participants will be able to explain how the studies discussed support the current thinking that tinnitus and hyperacusis have a central origin involving multiple brain regions.
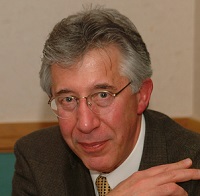
Richard Salvi
1. Thanks for joining me again for more discussion on tinnitus - I learned so much from last month's 20Q. Do you remember where we left off?
I was discussing research that has shown that somatic tinnitus is the rule rather than exception. Roughly 70% of tinnitus patients can make their tinnitus louder or quieter by movements of the head, neck, jaw, tongue and shoulders.
2. How could those movements possibly alter the activity in the auditory cortex?
As auditory neuroscientists, we are mainly concerned with the way in which the different dimensions of sound (e.g., intensity, frequency and time) activate specific regions of the peripheral or central auditory system. We become “auditory-centric” and ignore the fact that most regions of the central auditory pathway receive inputs and send outputs to many nonauditory centers involved with emotion, memory, body position, vision, touch -- and likely many other regions. By integrating many different types of information, we are able to carry out complex activities such as turning our head, torso and eyes towards a loud warning sound that induces fear or anxiety and increases our heart rate. In our evaluation of patients with somatic tinnitus, we observed that movements of the face, head, jaw, tongue, neck and shoulders - innervated by cranial nerves V, VII, XI and cervical spinal nerves - could modulate tinnitus loudness (Simmons, Dambra, Lobarinas, Stocking, & Salvi, 2008).
3. Is there other research supporting this?
Considerable. For example, work by Shore and colleagues (Basura, Koehler, & Shore, 2012) has shown that the trigeminal nerve (cranial nerve 5) can modulate sound evoked activity in the cochlear nucleus in the auditory brainstem; the trigeminal inputs to the cochlear nucleus become more robust as a result of cochlear hearing. The auditory cortex, as its name implies, is predominantly an auditory processing area. However, after profound bilateral deafening the auditory cortex no longer responds to sound because its acoustic inputs have been eliminated. Remarkably, Allman and coleagues (Allman, Keniston, & Meredith, 2009) found the auditory cortex “shifted its allegiance” to the somatosensory system a few weeks after deafening. Touching a deaf ferret on the face, neck or shoulder, but not elsewhere, induced robust neural responses in the auditory cortex.
4. Interesting. Let’s talk about tinnitus treatment. I hear people mention the use of the drug lidocaine?
Lidocaine, also known as xylocaine and lignocaine, is a medication sometimes used to numb tissue in a specific area, and also to treat ventricular tachycardia. When used as an antiarrhythmic medication, lidocaine works by blocking sodium channels, which decreases the rate of contractions of the heart. As a numbing agent, local neurons cannot signal the brain. The drug has been around since the late 1940s, and yes, it has been used in tinnitus research dating back to the 1980s.
5. Has your group studied the effects of lidocaine on tinnitus?
Yes, we used lidocaine to study the neural generator of tinnitus, and that research made me aware of my scientific tunnel vision. When we read the scientific literature on the use of intravenous lidocaine to suppress tinnitus, my colleagues and I confined our readings to the otologic and audiologic literature. The vast majority of publications in this domain stated that lidocaine suppressed tinnitus. Consequently, when we carried out our PET imaging study with tinnitus patients and normal hearing controls, we assumed that lidocaine would suppress the phantom sound in tinnitus patients and have no effect on control subjects. Our predictions were overturned by the results (Reyes et al., 2002). We were shocked to find that lidocaine induced tinnitus in some of our control subjects, made it louder in some tinnitus patients, and in other patients it reduced the loudness of their tinnitus. Had we read the cardiology literature, not just the otology publications, we would have realized that lidocaine can induce or exacerbate tinnitus.
6. Did that finding prevent you from obtaining meaningful results from the study?
Not at all. To deal with the bidirectional effects of lidocaine on tinnitus, we performed an analysis of covariance on the PET imaging data. This was done in order to identify the regions of the brain where neural activity changed when tinnitus loudness increased or decreased. The change in tinnitus loudness was linked to significant change in neural activity in the right auditory association cortex. Increases in tinnitus loudness were associated with an increase in neural activity, while decreases in tinnitus loudness were associated with decreased neural activity. The neural activity associated with the lidocaine-induced changes in tinnitus were again confined to just one auditory cortex, consistent with a central origin of tinnitus rather than a cochlear origin. Results from our previous studies of somatic tinnitus also pointed to a central origin.
7. I know you’ve done some research with animals. Do they have subjective tinnitus too?
Yes, animal models make behavioral responses indicating that they experience tinnitus. In animal research, however, we induce the tinnitus.
8. How can animals tell us if they are experiencing a phantom sound?
The lack of behavioral techniques to assess tinnitus in animals was a major impediment to the field until a major breakthrough by Jastreboff. He developed a behavioral lick-suppression technique to assess tinnitus in rats (Jastreboff, Brennen, & Sasaki, 1989). His behavioral model was validated using high doses of sodium salicylate, the active ingredient in aspirin which consistently induces tinnitus in humans when taken in large amounts. Since then, a large number of new behavioral techniques have been developed to assess tinnitus induced by noise exposure or salicylate. With the exception of the gap-startle technique, all of the methods rely on positive or negative reinforcement techniques as detailed in our recent review paper (Hayes, Radziwon, Stolzberg, & Salvi, 2014).
9. Could you give an example of how this might work?
Sure. In general, animals are trained to make one type of response if they hear a sound (e.g., press the left bar to get a reward) and a second type of response (e.g., press the right bar to get a reward) in the absence of sound stimulation (i.e., quiet) (Stolzberg et al., 2013). Once the animals can correctly distinguish between a real sound(s) and quiet, researchers will then try to induce tinnitus with a high dose of salicylate (or noise exposure) to induce tinnitus. If the noise or drug treatment induces the phantom sound of tinnitus, the animal will shift its behavior on quiet trial; instead of pressing the right bar on quiet trials it will begin to press the left bar, which is associated with sound stimulation. The switch in behavior on quiet trials is interpreted as evidence that the animal is hearing a phantom sound.
10. You indicated in our talk last month that hyperacusis is often associated with tinnitus. How can hyperacusis be measured in an animal model?
In order to measure hyperacusis, you really need to develop a behavioral method for assessing the growth of loudness. Several decades ago, psychophysicists were able to show that humans responded more rapidly (e.g., pressing a button) as the intensity of a sound increased. By measuring reaction time-intensity functions and psychophysical loudness growth functions, they were able to show that reaction time was closely correlated to loudness (Marshall & Brandt, 1980). Animal psychophysicists subsequently borrowed this technology and used it to show that reaction time-intensity functions became much steeper after an intense noise exposure. However, the reaction times at high intensity were typically equal to, but never shorter than, those measured before the exposure. In other words, the reaction time-intensity functions were recruitment-like, but there was no evidence of hyperacusis (shorter than normal reaction times) until recently.
11. So has any animal research shown the presence of hyperacusis?
It has—we looked at this a few years ago in our lab. Since high doses of salicylate consistently induced tinnitus in our behavioral models, we hypothesized that it might also induce hyperacusis. To test this hypothesis, we first measured the baseline reaction time-intensity functions in rats. Afterwards, we administered a high dose of salicylate and re-measured the reaction time-intensity functions shortly afterwards (Chen, Radziwon, Kashanian, Manohar, & Salvi, 2014). At low intensities, the reaction times were slightly longer than normal presumably due to the fact that salicylate induced a 20 dB hearing loss. However, as intensity-increased reaction times decreased and became shorter than normal, this was behavioral evidence that the sounds were now louder than they were before the drug was administered. The beauty of this approach is that each subject serves as its own control, ruling out individual differences in response speed as a confounding factor. This was the first study to demonstrate that high dose salicylate not only induces tinnitus, but also hyperacusis. We now have a robust and reliable behavioral method for inducing hyperacusis.
12. So high doses of salicylate induces tinnitus and hyperacusis. What effect does salicylate have on the cochlea?
It has been known for some time that high doses of salicylate are ototoxic. This is reflected as a reduction in distortion product otoacoustic emission, indicative of impaired outer hair cell function. In addition, the amplitude of the compound action potential (CAP), which reflects the neural output of the cochlea, is substantially decreased at all intensities and the CAP threshold is increased. The important point to note is that there is a substantial decrease in neural activity flowing from the cochlea to the central auditory system.
13. Does the salicylate also affect neural activity at higher levels of the auditory pathway?
Recall that I mentioned earlier that tinnitus and hyperacusis likely involve the central nervous system, so we would expect that it does. When we made electrophysiological recordings at higher levels of the auditory pathway, we continued to observe thresholds shifts in the inferior colliculus, medial geniculate body and auditory cortex of the same magnitude as those measured at the cochlea. However, at suprathreshold intensities we found that neural responses at the inferior colliculus were essentially normal, while those measured at the medial geniculate and auditory cortex were substantially larger than normal. The loss of neural activity from the cochlea (hypoactivity) resulted in suprathreshold hyperactivity in the central auditory pathways. The degree of hyperactivity increased between the inferior colliculus and auditory cortex. The central auditory pathway seems to have a “volume control” to compensate for a reduced output from the cochlea. What is remarkable is that this increase in central gain occurred within a few hours. The enhanced central gain associated with high dose salicylate could be the neural correlate of our behavioral measures of hyperacusis (G.D. Chen et al., 2014).
14. Did you study the effects of regions of the brain outside of the auditory cortex?
Some regions of the brain such as the amygdala attach emotional significance (e.g., fear, anxiety) to auditory and other forms of sensory stimulation. Although the amygdala lies outside the classically defined auditory pathway, some portions of the amygdala respond to sound stimulation and therefore could be affected by cochlear hearing loss. To test this hypothesis, we placed recording electrodes into the lateral amygdala and made electrophysiological measurements from the amygdala before and several hours after administering a high dose of salicylate known to induce tinnitus and hyperacusis. High dose salicylate caused the sound evoked threshold in the lateral amygdala to increase by the same amount as those in the cochlea, indicating that the hearing loss was cochlear in origin. However, supra-threshold responses were much larger than normal following salicylate treatment. The sound evoked hyperactivity in the amygdala would presumably intensify the negative emotional valence attached to a real sound or the phantom sound of tinnitus.
15. What about other brain areas?
We were cognizant of the fact that salicylate could be affecting multiple regions of the brain. Identifying all the possible regions that could be affected using our electrophysiological methods would be extremely time consuming and labor intensive. Therefore, we decided to use functional magnetic resonance imaging (fMRI) to carry out a broad survey of the entire brain. To accomplish this, we measured the amplitude of low frequency fluctuation (ALFF) of the fMRI BOLD signal at different points (voxels) within the brain. The amplitude of the ALFF BOLD signal fluctuates at a low frequency (<0.1 Hz); these fluctuations presumably reflect the spontaneous fluctuations in neural activity. To determine which regions of the brain were affected, we treated one group of rats with a high dose of salicylate sufficient to induce tinnitus and hyperacusis, and the other group (controls) were given saline. Afterwards, we identified the regions of the brain where there was a significant increase or decrease in the ALFF signal (Y.C. Chen et al., 2015).
16. What were the findings?
Consistent with our electrophysiological data, we found that ALFF increased in the inferior colliculus, medial geniculate, auditory cortex and amygdala. The high degree of correspondence gave us confidence in the ALFF measurement technique. Interestingly, ALFF identified some additional areas of increased activity, namely the reticular formation, which is involved in arousal, and the parafloccular lobe of the cerebellum, previously implicated in gating tinnitus. We also observed a decrease in the ALFF in the hippocampus, important for memory and spatial navigation.
17. Earlier you mentioned that higher portions of the central nervous system are highly interconnected. Is that something you were able to assess?
Powerful new research tools have been developed to look at the patterns of functional connectivity in the brain. As I mentioned earlier, the BOLD fMRI signal fluctuates spontaneously in the resting state. If two regions of the brain are connected and communicating with one another, then the spontaneous fluctuation in the BOLD signal from one point in the brain (voxel) is likely to be synchronized (correlated) with the other, i.e., the two regions are functionally connected with one another. We used a seed-based Functional Connectivity (FC) approach to determine if high dose salicylate altered the functional connectivity pattern (Y.C. Chen et al., 2015).
18. So, did the salicylate treatment alter brain connectivity?
We found a large increase in FC in an auditory network consisting of the auditory cortex, medial geniculate and inferior colliculus. In addition, we found a large increase in functional connectivity between the auditory cortex and two other structures, the reticular formation and parafloccular lobe of the cerebellum. In addition, we found a large increase in functional connectivity between the medial geniculate and hippocampus and the inferior colliculus and hippocampus.
19. You’ve talked about a lot of different research. Have you developed a scientific framework for interpreting all of the data from your animal studies?
As you’ve noticed, we have considerable behavioral, electrophysiologic and fMRI data from several different research projects. From these studies, we have come up with a couple key ideas. First, cochlear damage from ototoxic drugs or intense noise invariably reduces the neural output from the cochlea. Paradoxically, the reduced input “jacks up” sound evoked activity at many points along the central auditory pathway as well as some sites outside the classically defined auditory network (Auerbach, Rodrigues, & Salvi, 2014). By increasing the gain, the central auditory pathway attempts to compensate for the lack of input from the cochlea. The increase in central gain likely accounts for hyperacusis, loudness recruitment and possibly tinnitus. Second, salicylate-induced hearing loss, tinnitus and hyperacusis give rise to change in multiple brain regions. Hearing, interpreting and reacting to a real sound or phantom sound requires a brain network to: arouse us to changes in the environment (reticular activating system); determine if a sound is dangerous (amygdala); identify where the sound is located within the environment (hippocampus); consider if we have heard the sound before (hippocampal memory); evaluate how loud the sound is (auditory cortex, medial geniculate, inferior colliculus); and, decide what motor planning activity needs to be carried out to move smoothly and quickly away from an acoustic signal that signals danger (cerebellum).
20. Any final comments?
We hear with our brain, not just with the cochlea. Patients with debilitating tinnitus and hyperacusis react to phantom sounds and real sounds in a complex manner involving many brain regions with different functions. Although the brain networks responsible for tinnitus and hyperacusis may have some common features, the components of the network and their relative weights would be expected to account for the unique characteristics of each patient. The rapid advances in functional and structural brain imaging may usher in a new era of individualized tinnitus and hyperacusis assessment far beyond what we currently can imagine.
References
Allman, B.L., Keniston, L.P., & Meredith, M.A. (2009). Adult deafness induces somatosensory conversion of ferret auditory cortex. Proc Natl Acad Sci U S A, 106(14), 5925-5930. doi:10.1073/pnas.0809483106
Auerbach, B.D., Rodrigues, P.V., & Salvi, R.J. (2014). Central gain control in tinnitus and hyperacusis. Front Neurol, 5, 206. doi:10.3389/fneur.2014.00206
Basura, G.J., Koehler, S.D., & Shore, S.E. (2012). Multi-sensory integration in brainstem and auditory cortex. Brain Res, 1485, 95-107. doi:10.1016/j.brainres.2012.08.037
Chen, G. D., Radziwon, K. E., Kashanian, N., Manohar, S., & Salvi, R. (2014). Salicylate-induced auditory perceptual disorders and plastic changes in nonclassical auditory centers in rats. Neural Plast, 2014, 658741. doi:10.1155/2014/658741
Chen, Y C., Li, X., Liu, L., Wang, J., Lu, C.Q., Yang, M., . . . Teng, G.J. (2015). Tinnitus and hyperacusis involve hyperactivity and enhanced connectivity in auditory-limbic-arousal-cerebellar network. Elife, 4, e06576. doi:10.7554/eLife.06576
Hayes, S.H., Radziwon, K.E., Stolzberg, D.J., & Salvi, R.J. (2014). Behavioral models of tinnitus and hyperacusis in animals. Front Neurol, 5, 179. doi:10.3389/fneur.2014.00179
Jastreboff, P.J., Brennen, J.F., & Sasaki, C.T. (1989). An animal model of tinnitus. Laryngoscope, 98, 280-286.
Marshall, L., & Brandt, J.F. (1980). The relationship between loudness and reaction time in normal hearing listeners. Acta Otolaryngol, 90(3-4), 244-249. Retrieved from https://www.ncbi.nlm.nih.gov/pubmed/7468185
Reyes, S. ., Salvi, R. ., Burkard, R. ., Coad, M. ., Wack, D. ., Galantowicz, P. ., & Lockwood, A. . (2002). Brain imaging of the effects of lidocaine on tinnitus. Hear Res, 171(1-2), 43-50. Retrieved from https://www.ncbi.nlm.nih.gov/pubmed/12204348
Simmons, R., Dambra, C., Lobarinas, E., Stocking, C., & Salvi, R. (2008). Head, neck and eye movements that modulate tinnitus. Sem. Hear., 29, 361-370.
Stolzberg, D., Hayes, S.H., Kashanian, N., Radziwon, K., Salvi, R.J., & Allman, B.L. (2013). A novel behavioral assay for the assessment of acute tinnitus in rats optimized for simultaneous recording of oscillatory neural activity. J Neurosci Methods, 219(2), 224-232. doi:10.1016/j.jneumeth.2013.07.021
Citation
Salvi, R. (2016, March). 20Q: Tinnitus. AudiologyOnline, Article 16622. Retrieved from https://www.audiologyonline.com.