From the Desk of Gus Mueller
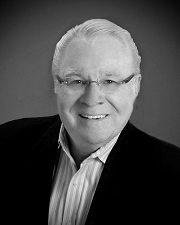
Cochlear synaptic transmissions are pretty important. They allow for representation of information that was contained in a given stimulus to be coded in the neural response. But, these transmissions can be lost, which is commonly referred to as cochlear synaptopathy—which has become an intriguing area of research in recent years.
What causes their disappearance? The most common causes are believed to be noise exposure and aging. Some research has suggested that synapses also can be lost due to exposure to some ototoxic drugs and genetic mutations. The synaptic loss can occur before significant hair cell damage is present, and consequently, is not typically observed by routine audiologic testing (i.e., pure tones and speech recognition in quiet). For this reason, it sometimes has been termed “hidden hearing loss.” That is, the patient might have a complaint of not being able to understand speech effectively in background noise, yet pure tone thresholds are normal.
While there are some audiologic tests that might indicate that cochlear synaptopathy exists, this diagnosis can be murky, as there often is some accompanying hair cell damage. It's an interesting topic to discuss here at 20Q, and we did just that last year when Sharon Kujawa was our guest author—you can find that article here. Once really is not enough for this topic, so we have another expert to give us a slightly different view on “hidden hearing loss.”
Colleen Le Prell, PhD, is Professor, The University of Texas at Dallas, School of Behavioral and Brain Science. She also is the Program Head for the AuD training program at UTD—consistently rated one of the top training programs in the U.S. She is Associate Editor for the International Journal of Audiology, and in addition to her many journal publications, has edited three books.
Dr. Le Prell is internationally known for her work in the area of hearing loss prevention and is the past president of the National Hearing Conservation Association. Her work includes the identification of cell death pathways activated by noise, and assessment of therapeutic agents that prevent cell death and hearing loss. Her research spans the scientific translational spectrum — from basic scientific investigations that identify promising therapeutic agents for the prevention of noise-induced hearing loss, to the assessment of these agents in clinical trials. In this excellent 20Q article, Colleen explains how her research relates to cochlear synaptopathy, and how physiologic findings link to clinical audiology.
Gus Mueller, PhD
Contributing Editor
Browse the complete collection of 20Q with Gus Mueller CEU articles at www.audiologyonline.com/20Q
20Q: Hearing Science "Hide and Seek" - Can Audiologists Diagnose Hidden Hearing Loss?
Learning Outcomes
After this course, readers will be able to:
- Define hidden hearing loss
- Explain the findings of key research studies in the areas of cochlear synaptopathy and noise-induced hearing loss in animal models and humans
- Describe the contribution of select audiological tests in the differential diagnosis of cochlear synaptopathy
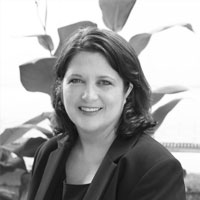
Colleen Le Prell
1. I hear lots of people talking about hidden hearing loss these days. Can you tell me what this is?
You are right on this one – it seems like everyone is talking about this. Hidden hearing loss is a term used to describe permanent auditory nerve synaptic pathology “hidden” behind normal hair cell counts, which is accompanied by a decrease in ABR Wave–I amplitude. We use the term “hidden” to indicate that this Wave-I amplitude decrease is hidden behind normal threshold sensitivity (Kujawa & Liberman, 2006; 2009). The term hidden hearing loss was originally proposed by Schaette and McAlpine (2011) to specifically refer to decreased Wave I amplitude. However, this term is also increasingly used to refer to auditory dysfunction that is speculated to accompany cochlear synaptopathy and accompanying decreased Wave I amplitude (Kujawa & Liberman, 2015).
2. What do you mean by “speculated to accompany?”
The most commonly hypothesized functional effect of noise-induced neuropathic damage is difficulty understanding speech in noisy environments (Kujawa & Liberman, 2009; Lin, Furman, Kujawa, & Liberman, 2011; Makary, Shin, Kujawa, Liberman, & Merchant, 2011; see also the detailed discussions by Plack, Barker, & Prendergast, 2014; Plack et al., 2016; Pienkowski, 2017). Confirming this in humans has been challenging, and a number of research teams around the world are trying to answer important questions about differential diagnosis of this pathology. This is a topic of interest in our research laboratory, as well as many others. Regardless of whether speech in noise deficits are related to cochlear synaptopathy or other pathology, this is an important patient complaint. If we better understood risk factors for these complaints, we could improve our guidance on the prevention of the pathologies that lead to these issues.
3. What do we know about relationships between noise exposure, cochlear synaptopathy, and hearing in noise?
Much of the data is from animal models. Recently, we explored the effects of a noise-induced cochlear synaptopathy on a signal in noise detection test in a rat model. We documented poorer function on the signal in noise test when rats were tested before and after noise exposure, at least in the most difficult listening conditions (poorest signal to noise ratios) (Lobarinas, Spankovich, & Le Prell, 2017). However, deficits on the signal in noise test were only induced in those rats that had previously had a large TTS (40-50 dB, 24 hours post noise), and the deficits were limited to the subset of frequencies at which a permanent noise-induced decrease in ABR Wave I amplitude was measured after the TTS resolved. In addition, in a chinchilla study in which a loss of inner hair cells (IHCs) was induced using carboplatin, IHC loss compromised hearing in noise (Lobarinas et al., 2016). In that study, once loss of IHCs reached about 60%, thresholds for the detection of tones in noise increased by 5-10 dB, and masking functions were broadened, indicating that off-frequency listening was poorer.
4. What about human testing? I’ve had patients with normal hearing complain about problems hearing in noise.
That is a million dollar question. Given the animal data, it certainly seems reasonable to hypothesize that cochlear synaptopathy could contribute to deficits hearing in noise. A key challenge in differentially attributing these kinds of patient complaints to cochlear synaptopathy is how to weight the probabilities of outer hair cell (OHC) pathology and cochlear synaptopathy. Based on a model of the effects of synapse loss on basic perceptual tasks, it seems that cochlear synaptopathy would have to be quite severe to result in perceptual issues. Oxenham (2016) specifically suggested that the effects of 50% loss of synapses would be barely measurable on a variety of “just noticeable difference” tests. On the other hand, deficits in patients diagnosed with auditory neuropathy do suggest that increasingly significant pathology within the ascending neural pathway is likely to compromise speech in noise performance.
5. Is auditory neuropathy related to cochlear synaptopathy?
There are multiple etiologies of auditory neuropathy, which is now termed Auditory Neuropathy/Auditory Dys-synchrony (AN/AD). The classic profile of AN/AD is intact OHC function as measured by otoacoustic emissions (OAEs) in combination with an abnormal or absent auditory brainstem response (ABR), indicating aberrant neural responses to acoustic stimuli. The specific pathology likely varies from patient to patient, however, patients may have either pre- or post-synaptic damage, and post-synaptic damage may be at the nerve terminal or may involve the proximal auditory nerve (Starr, Sininger, & Pratt, 2000; Santarelli, Starr, Michalewski, & Arslan, 2008). Given the wide range of potential pathologies, there is not surprisingly a wide range of functional deficits, varying from deafness in the most extreme cases, to fluctuating hearing sensitivity, intact hearing but poor speech comprehension, or intact hearing with difficulties in noise in less affected cases (for discussion see Sininger, 2008).
6. Do I have this correct - patients with intact OHCs, at least as measured using OAEs, but disrupted ABR morphology can have speech in noise difficulties?
Absolutely. Starr and colleagues (Starr, Picton, Sininger, Hood, & Berlin, 1996; Starr, Sininger, & Pratt, 2000) described patients with AN as having normal hearing thresholds but difficulties processing speech. Impaired temporal processing (Zeng, Oba, Garde, Sininger, & Starr, 1999; Michalewski, Starr, Nguyen, Kong, & Zeng, 2005) and impaired loudness adaptation (Wynne et al., 2013) have also been reported in patients with AN (for review of additional data, see Le Prell & Lobarinas, 2016). The poorer temporal processing is often accompanied by a variety of other deficits, including pitch discrimination at low frequencies, temporal integration, gap detection, temporal modulation detection, backward and forward masking, signal detection in noise, binaural beats, and sound localization using interaural time differences (Zeng, Kong, Michalewski, & Starr, 2005). Given that clinical AN/AD includes intact OAEs in combination with absent or grossly abnormal ABR, Kujawa & Liberman (2015) specifically pointed to patients with AN/AD as examples of a human condition consistent with their results in animals.
7. You mentioned OHC loss might also influence speech in noise test outcomes?
I did, and this is a major issue because damage to the OHC population is often observed in parallel with neural or synaptic pathologies (for a detailed review, see Hickox, Larsen, Heinz, Shinobu, & Whitton, 2017). Measurements of auditory nerve discharge in response to vowel sounds (in animal models) (Bruce, Sachs, & Young, 2003) and data from human participants both suggest OHC damage may directly contribute to speech-in-noise deficits (see, for example, Leger, Moore, & Lorenzi, 2012; Summers, Makashay, Theodoroff, & Leek, 2013; Hoben, Easow, Peyzner, & Parker, 2017). Unless we are able to exclude both conductive hearing loss and damage to the OHCs, we cannot be confident that deficits first emerge as a consequence of a compromised neural pathway (which could arise from damage to the IHCs, loss of the synaptic connections between the IHCs and the auditory nerve fibers, or pathology of the auditory nerve itself).
8. This then means that diagnostic OAE tests are a critical component of our test battery?
This is true. OAEs are now routinely used across clinics as a tool for gaining insight into OHC function, as they provide good insight into OHC function. Diagnostic OAE protocols are critical, with careful inspection of response amplitude; completing a pass/fail screening test is not adequate for differential diagnosis. But, OAEs do also have some shortcomings that we need to keep in mind when differentiating potential OHC loss from potential cochlear synaptopathy. Specifically, normal DPOAEs can be recorded even in the presence of OHC damage and thus normal DPOAEs do not necessarily imply the OHC population is not damaged (Subramaniam, Henderson, & Spongr, 1994; Subramaniam, Salvi, Spongr, Henderson, & Powers, 1994; Chen & Fechter, 2003). In other words, it is possible to obtain normal OAEs even in the presence of OHC damage, reducing confidence in assumptions that the OHC population has not been subtly damaged in patients with complaints. We know that OHCs are particularly vulnerable so it is important to consider the potential for subtle pathology even when OAEs appear to be within normal limits.
Now, I realize that it might not be possible or even appropriate to simply conduct OAEs on all your patients. What I would suggest is diagnostic DPOAE testing be completed in those cases where audiometric thresholds are within normal limits, but the patient's complaints suggest difficulties in environments with a poor signal-to-noise ratio (SNR).
9. Let’s shift gears a little and talk about speech in noise tests. What test (or tests) are being used right now?
One of the tests being used in research studies, that is readily available to clinicians, is the Quick Sentences in Noise (QuickSin). This is a sentence-based test, with sentences presented at 70-dB HL while the level of the four-talker babble background is adjusted from +25 dB SNR (easiest) to 0 dB SNR (hardest) in 5 dB SNR steps (Killion, Niquette, Gudmundsen, Revit, & Banerjee, 2004); McArdle and Wilson, 2006). Another test is the Words in Noise (WIN) test. During testing with the WIN, Northwestern University List #6 (NU-6) words are presented in multiple-talker babble, with a female speaker against a background babble that includes six competing female voices. The babble is set at 80 dB SPL and the target word levels decrease from 104 dB SPL (24 dB SNR) to 80 dB SPL (0 dB SNR) in 4-dB decrements, with 5 words per condition (Wilson, Carnell, & Cieghorn, 2007; Wilson & McArdle, 2007). A test that incorporates spatial listening into the speech in noise test is the Listening in Spatialized Noise - Sentences High Cue Condition (LiSN-S). Two-talker masker noise is presented at 61 dB SPL, 90 degrees relative to the listener, and the target speech is delivered at 68 dB SPL, 0 degrees relative to the listener (for discussion see Yeend, Beach, Sharma, & Dillon, 2017; Valderrama et al., 2018). Another test incorporating spatial listening is the Coordinate Response Measure (CRM) test, during which participants must listen for a color and number in the presence of 80 dB SPL background noise. Masker noise is delivered via two speakers each at 60 degrees relative to the listener, and the target speech is delivered at 0 degrees relative to the listener, with the level varied in 2-dB increments to determine threshold (for additional detail, see Prendergast et al., 2017b). To increase the difficulty of the test, others are developing custom tests. For example, Liberman and colleagues (2016) developed a difficult custom task, including the identification of NU-6 words at an SNR of 0 dB or 5 dB, with or without digital time compression (45% or 65% of original duration), and with 0.3 sec reverberation added.
10. Comparing across tests, what would be the best test for revealing cochlear synaptopathy in my patients?
I want to be careful here with our wording here. Since OHC pathology can contribute to hearing in noise difficulties, we need to be careful not to assume that all deficits on a speech in noise test are a function of cochlear synaptopathy. For that reason, I refer to speech in noise deficits as speech in noise deficits; I do not term these “hidden hearing loss”. They are not hidden to the patient, and they are not hidden to us, as long as we take the time to measure them. With that said, I wish I could give you a good answer on the “best” tests but I really can’t. Not only are different research teams using different speech in noise tests, but they are also studying different populations, and measuring noise exposure history using different techniques. Trying to allocate differences in results to differences in populations vs differences in protocols is difficult. So, let me just give you an overview of what’s been found with respect to speech in noise deficits as a function of noise exposure.
In studies by Fulbright et al. (2017), Grinn et al. (2017), and Le Prell and colleagues (2018), the WIN test was used, with no relationships between WIN threshold and noise exposure history. In studies by Yeend et al. (2017) and Valderrama et al. (2018), the LiSN-S was used, and neither study reported relationships between LiSN-S performance and noise history. One of the most carefully controlled investigations to date is that of Guest and colleagues (Guest, Munro, Prendergast, Millman, & Plack, 2018), who specifically recruited individuals self-reporting deficits perceiving speech in noise. They verified deficits using the CRM test, and assessed relationships between lifetime noise exposure history, self-reported and verified speech-in-noise deficits, and ABR Wave-I amplitude, with no statistically significant relationships detected. Prendergast et al. (2017b) had similarly failed to detect statistically significant relationships between noise exposure history and the CRM test, as well as a variety of other tests including the digit triplet test, during which three spoken digits with varied sound levels were presented sequentially in background noise at 40 or 80 dB SPL. No statistically significant relationships emerged during a battery of other tests, including frequency difference limens, intensity difference limens, interaural phase difference discrimination, amplitude modulation detection thresholds, localization and musical consonance task were also assessed.
Grose, Buss, and Hall (2017) approached this question in a very different way. Instead of using interviews and/or surveys to quantify noise exposure for use in correlation or regression analyses, they recruited participants with a history of frequent concert attendance (defined as at least 25 concerts within the past year, and at least 40 within the past two years) and compared them to participants with minimal concert attendance (averaging four concerts in the previous two years). Using BKB sentences with the target words filtered from 1000-2000 Hz or 3000-6000 Hz presented at 60 and 80 dB SPL, thresholds were determined for the target words presented against a corresponding speech-shaped noise with an equivalent spectral envelope with the background babble varied in 2 dB steps to determine threshold. No differences in performance on the BKB sentences were detected when those with a history of frequent concert attendance were compared to those with minimal concert attendance. Other psychoacoustic tests completed as part of the study by Grose et al. (2017) included temporal modulation detection, spectral modulation detection, and sensitivity to interaural phase, with no relationships with concert attendance detected.
11. Do you find it surprising that so few studies find hearing in noise deficits as a function of noise exposure history?
The results are fairly consistent with studies in animals. For example, in studies in which rodent subjects had a smaller TTS, averaging 20-30 dB TTS 24 hours post noise instead of the 40-50 dB TTS mentioned above, there generally has not been a measurable synaptic pathology (Hickox & Liberman, 2014; Fernandez, Jeffers, Lall, Liberman, & Kujawa, 2015; Jensen, Lysaght, Liberman, Qvortrup, & Stankovic, 2015). The participants in the studies by Prendergast et al. (2017b), Fulbright et al. (2017), Grinn et al. (2017), Yeend et al. (2017), Le Prell et al. (2018), Guest et al. (2018), and Valderrama et al. (2018) likely had not ever experienced acoustic trauma sufficient to result in the 40-50 dB TTS 24 hours post noise. In fact, they probably would not have experienced even a 20-30 dB TTS 24 hours post noise from any of the occupational or non-occupational exposures they had previously experienced. Granted, we do not know how directly results transfer from rodents to humans, but, we do know that as you move from rodents to primates, the amount of noise needed to induce cochlear synaptopathy increases (Valero et al., 2017). Thus, even the concert goers recruited by Grose et al. (2017) probably would not experience TTS sufficient to induce cochlear synaptopathy as TTS measured immediately after concerts or noisy clubs is commonly on the order of 10 dB (Opperman, Reifman, Schlauch, & Levine, 2006; Derebery, Vermiglio, Berliner, Potthoff, & Holguin, 2012; Ramakers, Kraaijenga, Cattani, van Zanten, & Grolman, 2016), with complete threshold recovery within 24 hours of the event likely for most attendees (Grinn et al., 2017).
12. Why has so much time and energy been invested in studying populations with limited risk?
You have to remember, before these studies we were completed, we did not know who would be at risk. Humans are exposed to intense sound on a routine basis, which may be very different from the single short-term exposure paradigms used in animals. Recreational activities including concerts, clubs, and sporting events, are loud; busses, subways, and other public transportation can be loud, and sometimes radios are played at high sound levels inside personal vehicles; mowing the lawn and using power tools for hobbies or home repair are loud as well. All of these activities are likely to be repeated on a regular basis, and the potential hazards of repeated noise exposure are virtually unexplored with respect to potential risk for cochlear synaptopathy. The rodent studies have not been designed to allow broad conclusions regarding safety as they typically include only a single exposure whereas human exposures are likely to be repeated. Given concerns about the hazards of the repetition of such exposure, in combination with other intense sound encountered on a regular basis, specific questions about the risk of synaptopathy in concert goers and music player users have been raised (Jensen et al., 2015; Liberman, 2015). In addition, there was the early report by Stamper and Johnson (2015a), who found statistically significant relationships between recreational noise exposure (in the past year) and ABR Wave I amplitude (used to infer potential cochlear synaptopathy), although these relationships were later found to be limited to females after re-analysis of the data adjusting for participant gender (Stamper & Johnson, 2015b).
13. Have there been other studies that have reported evidence of cochlear synaptopathy in humans?
Three studies have had a particularly high impact. The first of these is the report by Stamper and Johnson (2015a), mentioned earlier. This early report describing statistically significant relationships between recreational noise exposure (in the past year) and ABR Wave I amplitude (used to infer potential cochlear synaptopathy) stimulated a search for functional deficits in similar populations, with similarly limited noise exposure. The relationships between ABR Wave I amplitude and noise exposure history within this sample were later found to be limited to females (Stamper & Johnson, 2015b). Other studies since then, using similar recruiting and testing protocols, have failed to detect statistically significant relationships between noise exposure history and ABR Wave I amplitude (Fulbright et al., 2017; Grinn et al., 2017; Spankovich, Le Prell, Hood, & Lobarinas, 2017). Additional studies recruiting more diverse participants (wider age ranges, with more careful accounting of lifetime noise exposure) have similarly failed to reveal statistically significant relationships (Prendergast et al., 2017a; Yeend et al., 2017; Guest et al., 2018). However, there is a new report by Valderrama et al. (2018) in which relationships between lifetime noise and ABR Wave I amplitude were detected, so these relationships are likely to remain a question of interest.
A second study which had a particularly high impact is that of Bramhall and colleagues (2017). Using different recruiting techniques to enroll different noise-exposed populations, they reported decreased ABR Wave I amplitudes which were attributed to recreational firearm use (civilians) or service-related firearm use (military personnel), as participants were distributed into four discrete groups based on use of firearms and exposure to other intense sound.
A third study with a particularly high impact is that of Liberman et al. (2016), who reported not only poorer speech-in-noise performance and poorer hearing at frequencies above 8000 Hz, but also a larger SP/AP (Summating Potential/Action Potential) ratio, which was driven by decreased SP amplitude in the higher-noise participants.
14. Is that high frequency hearing loss important?
Poorer high frequency hearing within the extended high frequency (EHF) range of 10 kHz and above has been reported in workers exposed to occupational noise (Hallmo, Borchgrevink, & Mair, 1995; Korres, Balatsouras, Tzagaroulakis, Kandiloros, & Ferekidis, 2008; Riga, Korres, Balatsouras, & Korres, 2010; Mehrparvar et al., 2014), personal audio system device users (Le Prell, Spankovich, Lobarinas, & Griffiths, 2013; Sulaiman, Husain, & Seluakumaran, 2015; Kumar, Upadhyay, Kumar, Kumar, & Singh, 2017), those with a history of musical training (Schmidt, Verschuure, & Brocaar, 1994; Goncalves, Lacerda, Zeigelboim, Marques, & Luders, 2013; Liberman et al., 2016), and those with acoustic trauma during military service (Balatsouras, Homsioglou, & Danielidis, 2005; Buchler, Kompis, & Hotz, 2012). In studies seeking evidence of noise-induced hidden hearing loss, EHF deficits have been reported in individuals with higher lifetime noise exposure (Prendergast et al., 2017a; Yeend et al., 2017), frequent concert goers (Grose et al., 2017), and the high risk population recruited by Liberman et al. (2016).
15. What does this mean for audiometric monitoring for occupational noise-induced hearing loss?
The federal noise regulations in the United States such as 29 CFR 1910.95 enforced by the US Occupational Safety and Health Administration (OSHA), and national regulations in other countries, include annual audiometric testing requirements so that the development of NIHL can be detected and additional hearing loss prevented. If a new hearing loss is detected (relative to the baseline audiogram on file for that worker), there will be typically some effort to complete a retest either immediately or after a noise-free interval to confirm the repeatability and/or permanence of the change. If the hearing loss meets certain criteria, it must be reported. Specifically, per 29 CFR 1904.10, hearing loss is required to be reported as an occupational noise injury when 1) a standard threshold shift (STS) is documented during annual audiometric testing (with STS defined as a permanent threshold change averaging 10-dB or more at the frequencies of 2, 3, and 4 kHz), 2) the average threshold at the 2, 3, and 4 kHz frequencies is 25 dB HL or poorer, and 3) exposure in the workplace may have caused or contributed to the hearing loss (OSHA, 1983). If cochlear synaptopathy were to develop as a consequence of exposure to occupational noise, the threshold-based STS strategies mandated by OSHA would not be sensitive to IHC injury, cochlear synaptopathy, or other hidden auditory nerve injuries (for additional discussion, see Kujawa & Liberman, 2009; Kujawa, 2014; Kujawa & Liberman, 2015; Liberman & Kujawa, 2017). The translational relevance of cochlear synaptopathy to noise-exposed workers is a topic that remains actively under discussion (see Dobie & Humes, 2017; Murphy & Le Prell, 2017).
16. Have you looked at the research literature on the topic of the cochlear synaptopathy in noise-exposed workers? What does it tell us?
Across studies reporting ABR data for diverse noise-exposed worker populations, there are multiple reports that the latency for Waves I, III, and/or V were delayed in workers exposed to noise, with a smaller number of studies measuring or reporting the amplitude for Waves I, III, or V (Attias & Pratt, 1984; Almadori et al.,1988; Chen, Chiang, & Chen, 1992; Donaldson & Ruth, 1996; Noorhassim, Kaga, & Nishimura, 1996; Xu, Vinck, De Vel, & van Cauwenberge, 1998; Thakur, Anand, & Banerjee, 2004; Karawani, Attias, Shemesh, & Nageris, 2015; Pushpalatha & Konadath, 2016). Although prolonged ABR waveform latencies were commonly reported across studies, most of these studies also reported that the noise-exposed worker cohorts had significant hearing loss. The presence of a permanent NIHL suggests OHC pathology accompanied any neuropathic change that might potentially be inferred from the ABR latency increases and other atypical ABR results. Decreased DPOAE amplitude is well documented in noise-exposed populations (Seixas et al., 2004; Korres et al., 2009; Seixas et al., 2012; Boger, Sampaio, & Oliveira, 2017). Although OHC deficits likely contributed to the observed changes in ABR morphology and timing, one might speculate that neural pathology contributed to delayed ABR latencies and reduced ABR amplitudes, even if permanent threshold deficits were caused by undocumented OHC loss. Although speculative, this interpretation is consistent with the conclusion by Hickox et al. (2017) that OHC loss and cochlear synaptopathy are likely to occur in parallel. Longitudinal studies of workers would be helpful in determining effects of noise on the auditory nerve in workers exposed to occupational noise.
17. As a clinician, what tests should I consider for the differential diagnosis of cochlear synaptopathy?
As per the Joint Audiology Committee Algorithm on Comprehensive Audiologic Assessment, the components of the assessment are dictated by patient need and may vary across patients. For differential diagnosis of cochlear synaptopathy, a careful patient history, otoscopy, and tympanometry, will be the basic starting point. Pure-tone air and bone conduction testing are warranted, to assess any audibility issues and distinguish conductive and sensorineural components. Pure-tone testing should include EHF audiometry given the reliable observation of high frequency hearing deficits in association with Wave I amplitude deficits. OAEs are critical, and given the availability of new equipment that facilitates testing at higher frequencies, high frequency OAEs should be considered in order to gain insight into the health of the basal cochlea. Although there is not yet sufficient evidence to advocate middle ear reflex testing for the purpose of diagnosing cochlear synaptopathy, this testing should be considered as part of the comprehensive battery as a cross-check of the audiogram and to gain insight into potential conductive, cochlear, and retro-cochlear pathologies (Jerger, Jerger, & Hall, 1979; Prasher & Cohen, 1993; Neary et al., 1996; although see also Hunter, Ries, Schlauch, Levine, & Ward, 1999). The amplitude of the middle ear muscle reflex has been reliably associated with cochlear synaptopathy in mouse models (Valero, Hancock, & Liberman, 2016; Valero, Hancock, Maison, & Liberman, 2018) generating interest in this test for use diagnosing cochlear synaptopathy. Specifically, the fact that middle ear muscle reflexes are weak, or absent, in a subset of the general population (Flamme, Deiters, Tasko, Ahroon, 2017; McGregor et al., 2018), has lead several investigators to suggest that noise exposure resulting in cochlear synaptopathic injury could provide one explanation for individual variability in human participants (Wojtczak, Beim, & Oxenham, 2017; McGregor et al., 2018). The last element of the test battery is, of course, auditory evoked electrophysiological testing.
18. Are reductions in ABR Wave I a reliable marker for cochlear synaptopathy?
There is indeed a strong correlation between cochlear synaptopathy and the amplitude of Wave-I of the ABR in rodent ears in which OHC function is intact (Sergeyenko, Lall, Liberman, & Kujawa, 2013), but human patients and participants have diverse risk factors for OHC loss and the OHC population may be damaged. Although the amplitude of Wave-I of the ABR serves as the gold standard within animal models, a number of alternative evoked potential metrics continue to be evaluated for potential use in human test batteries. When using the ABR, stimulus rates should probably be on the order to 11 to 21 per second as response amplitudes begin to decrease with higher stimulus presentation rates. Responses should be averaged across at least 1000-2000 presentations, with perhaps as many as 4000 presentations required if tests are completed at or near threshold, to reduce noise in the evoked responses (see Hall, 1992). ABR Wave I is repeatable and reliable within subjects, as is Wave V, although SP is less reliable from test to test (Prendergast et al., 2018).
19. I’m looking for a take-home message. Does cochlear synaptopathy occur in humans?
Keep in mind, the only way to confirm cochlear synaptopathy is with histology, so there are very few studies in which cochlear synaptopathy has been confirmed in humans. But, from the small number of studies to date, yes, it certainly appears that cochlear synaptopathy occurs in humans as a function of aging. Progressive age-related cochlear synaptopathy was observed in five human temporal bones from donors ranging in age from 54-89 years old (Viana et al., 2015). The observation of fewer synaptic connections in human temporal bones from older donors builds on earlier reports documenting age-related loss of auditory nerve fibers (Felder & Schrott-Fischer, 1995) and spiral ganglion cells (Makary et al., 2011). Cochlear synaptopathy in human temporal bones can be observed in parallel with OHC loss although IHC populations may remain relatively intact (Wu et al., 2018). Whether noise exposure contributed to any of the observed synapse and neural declines in these temporal bones is not clear, but, age-related cochlear synaptopathy in mice that have not been exposed to noise and do not have other risk factors for hearing loss is well documented (Sergeyenko et al., 2013), and the amplitude of Wave I of the ABR is highly correlated with synapse loss in aging mice (Sergeyenko et al., 2013).
20. All very interesting. Where can I go for more information?
Detailed reviews of the literature on noise exposure, cochlear synaptopathy, and permanent auditory nerve pathology clearly describe the potential for permanent pathology in the ascending neural pathway in the absence of noise-induced PTS (for reviews, see Plack, Barker, & Prendergast, 2014; Kujawa & Liberman, 2015; Hickox et al., 2017; Kobel, Le Prell, Liu, Hawks, & Bao, 2017; Liberman, 2017; Liberman & Kujawa, 2017; Barbee et al., 2018; Le Prell, 2018).
Acknowledgments
Funding for previously completed studies on temporary threshold shift in humans was provided by U01 DC 008423 from the National Institutes of Health – National Institute on Deafness and Other Communication Disorders (NIH-NIDCD), as well as contracts to the University of Florida awarded by Sound Pharmaceuticals, Inc. and Edison Pharmaceuticals, Inc. Support for the preparation of this manuscript was provided by the Emilie and Phil Schepps Distinguished Professorship in Hearing Science at the University of Texas at Dallas.
References
Almadori, G., Ottaviani, F., Paludetti, G., Rosignoli, M., Gallucci, L., D’Alatri, L., & Vergoni, G. (1988). Auditory brainstem responses in noise-induced permanent hearing loss. Audiology, 27, 36-41.
Attias, J., & Pratt, H. (1984). Auditory evoked potentials and audiological follow-up of subjects developing noise-induced permanent threshold shift. Audiology, 23, 498-508.
Balatsouras, D.G., Homsioglou, E., & Danielidis, V. (2005). Extended high-frequency audiometry in patients with acoustic trauma. Clin Otolaryngol, 30, 249-254.
Barbee, C.M., James, J.A., Park, J.H., Smith, E.M., Johnson, C.E., Clifton, S., & Danhauer, J.L. (2018). Effectiveness of auditory measures for detecting hidden hearing loss and/or cochlear synaptopathy: A systematic review. Semin Hear, 39, 172-209.
Boger, M.E., Sampaio, A.L.L. & Oliveira, C. (2017). Analysis of hearing and tinnitus in workers exposed to occupational noise. Int Tinnitus J, 20, 88-92.
Bramhall, N.F., Konrad-Martin, D., McMillan, G.P., & Griest, S.E. (2017). Auditory brainstem response altered in humans with noise exposure despite normal outer hair cell function. Ear Hear, 38, e1-e12.
Bruce, I.C., Sachs, M.B., & Young, E.D. (2003). An auditory-periphery model of the effects of acoustic trauma on auditory nerve responses. J Acoust Soc Am, 113, 369-388.
Buchler, M., Kompis, M., & Hotz, M.A. (2012). Extended frequency range hearing thresholds and otoacoustic emissions in acute acoustic trauma. Otol Neurotol, 33, 1315-1322.
Chen, G.D., & Fechter, L.D. (2003). The relationship between noise-induced hearing loss and hair cell loss in rats. Hear Res, 177, 81-90.
Chen, T.J., Chiang, H.C., & Chen, S.S. (1992). Effects of aircraft noise on hearing & auditory pathway function of airport employees. J Occup Med, 34, 613-619.
Derebery, M.J., Vermiglio, A., Berliner, K.I., Potthoff, M., & Holguin, K. (2012). Facing the music: pre- & postconcert assessment of hearing in teenagers. Otol Neurotol, 33, 1136-1141.
Dobie R.A., & Humes L.E. (2017). Commentary on the regulatory implications of noise-induced cochlear neuropathy. Int J Audiol, 56(sup1), 74-78.
Donaldson, G.S., & Ruth, R.A. (1996). Derived-band auditory brain-stem response estimates of traveling wave velocity in humans: II. Subjects with noise-induced hearing loss and Meniere's disease. J Speech Hear Res, 39, 534-545.
Felder, E. & Schrott-Fischer, A. (1995). Quantitative evaluation of myelinated nerve fibres and hair cells in cochleae of humans with age-related high-tone hearing loss. Hear Res, 91, 19-32.
Fernandez, K.A., Jeffers, P.W., Lall, K., Liberman, M.C., & Kujawa, S.G. (2015). Aging after noise exposure: acceleration of cochlear synaptopathy in "recovered" ears. J Neurosci, 35, 7509-7520.
Flamme, G.A., Deiters, K.K., Tasko, S.M., & Ahroon, W.A. (2017). Acoustic reflexes are common but not pervasive: evidence from the National Health and Nutrition Examination Survey, 1999-2012. Int J Audiol, 56, 52-62.
Fulbright, A.N.C., Le Prell, C.G., Griffiths, S.K. & Lobarinas, E. (2017). Effects of recreational noise on threshold and supra-threshold measures of auditory function. Semin Hearing, 38, 298-318.
Goncalves, C.G., Lacerda, A.B., Zeigelboim, B.S., Marques, J.M., & Luders, D. (2013). Auditory thresholds among military musicians: conventional and high frequency. CoDAS, 25, 181-187.
Grinn, S.K., Wiseman, K.B., Baker, J.A., & Le Prell, C.G. (2017). Hidden hearing loss? No effect of common recreational noise exposure on cochlear nerve response amplitude in humans. Front Neurosci, 11, 1-24.
Grose, J.H., Buss, E., & Hall, J.W., 3rd (2017). Loud music exposure and cochlear synaptopathy in young adults: Isolated auditory brainstem response effects but no perceptual consequences. Trends Hear, 21, 2331216517737417.
Guest, H., Munro, K.J., Prendergast, G., Millman, R.E., & Plack, C. (2018). Impaired speech perception in noise with a normal audiogram: No evidence for cochlear synaptopathy and no relation to lifetime noise exposure. Hear Res, 364, 142-151.
Hall, J.W.III. (1992). Handbook of auditory evoked responses. Boston: Allyn and Bacon.
Hallmo, P., Borchgrevink, H.M., & Mair, I.W. (1995). Extended high-frequency thresholds in noise-induced hearing loss. Scand Audiol, 24, 47-52.
Hickox, A.E., Larsen, E., Heinz, M.G., Shinobu, L., & Whitton, J.P. (2017). Translational issues in cochlear synaptopathy. Hear Res, 349, 164-171.
Hickox, A.E., & Liberman, M.C. (2014). Is noise-induced cochlear neuropathy key to the generation of hyperacusis or tinnitus? J Neurophysiol, 111, 552-564.
Hoben, R., Easow, G., Pevzner, S., & Parker, M.A. (2017). Outer hair cell and auditory nerve function in speech recognition in quiet and in background noise. Front Neurosci, 11, 157.
Hunter, L.L., Ries, D.T., Schlauch, R.S., Levine, S.C. & Ward, W.D. (1999). Safety and clinical performance of acoustic reflex tests. Ear Hear, 20, 506-514.
Jensen, J.B., Lysaght, A.C., Liberman, M.C., Qvortrup, K., & Stankovic, K.M. (2015). Immediate and delayed cochlear neuropathy after noise exposure in pubescent mice. PLoS One, 10, e0125160.
Jerger, S., Jerger, J., & Hall, J. (1979). A new acoustic reflex pattern. Arch Otolaryngol, 105, 24-28.
Karawani, H., Attias, J., Shemesh, R., & Nageris, B. (2015). Evaluation of noise-induced hearing loss by auditory steady-state and auditory brainstem-evoked responses. Clin Otolaryngol, 40, 672-681.
Killion, M.C., Niquette, P.A., Gudmundsen, G.I., Revit, L.J., & Banerjee, S. (2004). Development of a quick speech-in-noise test for measuring signal-to-noise ratio loss in normal-hearing and hearing-impaired listeners. J Acoust Soc Am, 116, 2395-2405.
Kobel, M., Le Prell, C.G., Liu, J., Hawks, J.W., & Bao, J. (2017). Noise-induced cochlear synaptopathy: Past findings and future studies. Hear Res, 349, 148-154.
Korres, G.S., Balatsouras, D.G., Tzagaroulakis, A., Kandiloros, D., & Ferekidis, E. (2008). Extended high-frequency audiometry in subjects exposed to occupational noise. B-ENT, 4, 147-155.
Korres, G.S., Balatsouras, D.G., Tzagaroulakis, A., Kandiloros, D., Ferekidou, E., & Korres, S. (2009). Distortion product otoacoustic emissions in an industrial setting. Noise Health, 11, 103-110.
Kujawa, S.G. (2014). Putting the ‘neural’ back in sensorineural: Primary cochlear neurodegeneration in noise and aging. Hear J, 67, 8.
Kujawa, S.G. & Liberman, M.C. (2006). Acceleration of age-related hearing loss by early noise exposure: evidence of a misspent youth. J Neurosci, 26, 2115-2123.
Kujawa, S.G. & Liberman, M.C. (2009). Adding insult to injury: cochlear nerve degeneration after "temporary" noise-induced hearing loss. J Neurosci, 29, 14077-14085.
Kujawa, S.G. & Liberman, M.C. (2015). Synaptopathy in the noise-exposed and aging cochlea: Primary neural degeneration in acquired sensorineural hearing loss. Hear Res, 330, 191-199.
Kumar, P., Upadhyay, P., Kumar, A., Kumar, S., & Singh, G.B. (2017). Extended high frequency audiometry in users of personal listening devices. Am J Otolaryngol, 38, 163-167.
Le Prell, C.G. (2018, in press). Effect of noise exposure on human auditory function: Hidden versus not-so-hidden hearing loss. In: S. Hatzopoulos, A. Ciorba & M. Krumm (eds.) Advances in audiology, speech pathology, and hearing science, Volume 1: Clinical protocols and hearing devices. New Jersey: Apple Academic Press.
Le Prell, C.G. & Lobarinas, E. (2016). Clinical and translational research: Challenges to the field. In C.G. Le Prell, E. Lobarinas, R.R. Fay & A.N. Popper (Eds.), Translational research in audiology & the hearing sciences (pp. 241-265), Springer handbook of auditory research. New York: Springer.
Le Prell, C.G., Siburt, H.W., Lobarinas, E., Griffiths, S.K., & Spankovich, C. (2018). No reliable association between recreational noise exposure and threshold sensitivity, distortion product otoacoustic emission amplitude, or word-in-noise performance in a college student population. Ear Hear, [Epub ahead of print DOI: 10.1097/aud.0000000000000575].
Le Prell, C.G., Spankovich, C., Lobarinas, E., & Griffiths, S.K. (2013). Extended high frequency thresholds in college students: effects of music player use and other recreational noise. J Am Acad Audiol, 24, 725-739.
Leger, A.C., Moore, B.C., & Lorenzi, C. (2012). Abnormal speech processing in frequency regions where absolute thresholds are normal for listeners with high-frequency hearing loss. Hear Res, 294, 95-103.
Liberman, M.C. (2015). Hidden hearing loss. Sci Am, 313, 48-53.
Liberman, M.C. (2017). Noise-induced and age-related hearing loss: new perspectives and potential therapies. F1000Research, 6, 927.
Liberman, M.C., Epstein, M.J., Cleveland, S.S., Wang H., & Maison, S.F. (2016). Toward a differential diagnosis of hidden hearing loss in humans. PLoS One, 11, e0162726.
Liberman, M.C., & Kujawa, S.G. (2017). Cochlear synaptopathy in acquired sensorineural hearing loss: Manifestations and mechanisms. Hear Res, 349, 138-147.
Lin, H.W., Furman, A.C., Kujawa, S.G., & Liberman, M.C. (2011). Primary neural degeneration in the guinea pig cochlea after reversible noise-induced threshold shift. J Assoc Res Otolaryngol, 12, 605-616.
Lobarinas, E., Salvi, R. & Ding, D. (2016). Selective inner hair cell dysfunction in chinchillas impairs hearing-in-noise in the absence of outer hair cell loss. J Assoc Res Otolaryngol, 17, 89-101.
Lobarinas, E., Spankovich, C., & Le Prell, C.G. (2017). Evidence of “hidden hearing loss” following noise exposures that produce robust TTS and ABR wave-I amplitude reductions. Hear Res, 349, 155-163.
Makary, C.A., Shin, J., Kujawa, S.G., Liberman, M.C., & Merchant, S.N. (2011). Age-related primary cochlear neuronal degeneration in human temporal bones. J Assoc Res Otolaryngol, 12, 711-717.
McArdle R.A., & Wilson, R.H. (2006). Homogeneity of the 18 QuickSIN lists. J Am Acad Audiol, 17, 157-167.
McGregor, K.D., Flamme, G.A., Tasko, S.M., Deiters, K.K., Ahroon, W.A., Themann, C.L., & Murphy, W.J. (2018). Acoustic reflexes are common but not pervasive: evidence using a diagnostic middle ear analyser. Int J Audiol, 57, S42-s50.
Mehrparvar A.H., Mirmohammadi S.J., Davari M.H., Mostaghaci M., Mollasadeghi A., Bahaloo, M., & Hashemi, S.H. (2014). Conventional audiometry, extended high-frequency audiometry, and DPOAE for early diagnosis of NIHL. Iran Red Crescent Med J, 16, e9628.
Michalewski, H.J., Starr, A., Nguyen, T.T., Kong, Y.Y., & Zeng, F.G. (2005). Auditory temporal processes in normal-hearing individuals and in patients with auditory neuropathy. Clin Neurophysiol, 116, 669-680.
Murphy, W.J., & Le Prell, C.G. (2017). Making sound waves: selected papers from the 2016 annual conference of the National Hearing Conservation Association. Int J Audiol, 56, 1-3.
Neary, W.J., Newton, V.E., Laoide-Kemp, S.N., Ramsden, R.T., Hillier, V.F., & Kan, S.W. (1996). A clinical, genetic and audiological study of patients and families with unilateral vestibular schwannomas. II. Audiological findings in 93 patients with unilateral vestibular schwannomas. J Laryngol Otol, 110, 1120-1128.
Noorhassim, I., Kaga, K., & Nishimura, K. (1996). Pure-tone audiometry and auditory brainstem responses in noise-induced deafness. Am J Otolaryngol, 17, 31-35.
Opperman, D.A., Reifman, W., Schlauch, R., & Levine, S. (2006). Incidence of spontaneous hearing threshold shifts during modern concert performances. Otolaryng Head Neck, 134, 667-673.
Occupational Safety and Health Administration (OSHA). (1983). 29 CFR 1910.95. Occupational noise exposure; Hearing conservation amendment; Final Rule, effective 8 March 1983.
Oxenham, A.J. (2016). Predicting the perceptual consequences of hidden hearing loss. Trends Hear, 20, 2331216516686768.
Pienkowski, M. (2017). On the etiology of listening difficulties in noise despite clinically normal audiograms. Ear Hear, 38, 135-148.
Plack, C.J., Barker, D., & Prendergast, G. (2014). Perceptual consequences of "hidden" hearing loss Trends Hear, 18. doi: 10.1177/2331216514550621
Plack, C.J., Leger, A., Prendergast, G., Kluk K., Guest, H., & Munro, K.J. (2016). Toward a diagnostic test for hidden hearing loss. Trends Hear, 20. doi: 10.1177/2331216516657466
Prasher, D. & Cohen, M. (1993). Effectiveness of acoustic reflex threshold criteria in the diagnosis of retrocochlear pathology. Scand Audiol, 22, 11-18.
Prendergast, G., Guest, H., Munro, K., Kluk, K., Leger, A., Hall, D.A.,…Plack, C.J. (2017a). Effects of noise exposure on young adults with normal audiograms I: Electrophysiology. Hear Res, 344, 68-81.
Prendergast G., Millman R.E., Guest H., Munro K.J., Kluk K., Dewey, R.S.,…Plack, C.J. (2017b). Effects of noise exposure on young adults with normal audiograms II: Behavioral measures. Hear Res, 356, 74-86.
Prendergast, G., Tu, W., Guest, H., Millman, R.E., Kluk, K., Couth, S.,…Plack, C.J (2018). Supra-threshold auditory brainstem response amplitudes in humans: Test-retest reliability, electrode montage and noise exposure. Hear Res, 364, 38-47.
Pushpalatha, Z.V., & Konadath, S. (2016). Auditory brainstem responses for click and CE-chirp stimuli in individuals with and without occupational noise exposure. Noise Health, 18, 260-265.
Ramakers, G.G.J., Kraaijenga, V.J.C., Cattani, G., van Zanten, G.A., & Grolman, W. (2016). Effectiveness of earplugs in preventing recreational noise-induced hearing loss: A randomized clinical trial. Jama Otolaryngol, 142, 551-558.
Riga, M., Korres, G., Balatsouras, D., & Korres, S. (2010). Screening protocols for the prevention of occupational noise-induced hearing loss: the role of conventional and extended high frequency audiometry may vary according to the years of employment. Med Sci Monit, 16, CR352-356.
Santarelli, R., Starr, A., Michalewski, H.J., & Arslan, E. (2008). Neural and receptor cochlear potentials obtained by transtympanic electrocochleography in auditory neuropathy. Clin Neurophysiol, 119, 1028-1041.
Schaette, R., & McAlpine, D. (2011). Tinnitus with a normal audiogram: physiological evidence for hidden hearing loss and computational model. J Neurosci, 31, 13452-13457.
Schmidt, J.M., Verschuure, J., & Brocaar, M.P. (1994). Hearing loss in students at a conservatory. Audiology, 33, 185-194.
Seixas, N.S., Kujawa, S.G., Norton, S., Sheppard, L., Neitzel, R., & Slee, A. (2004). Predictors of hearing threshold levels and distortion product otoacoustic emissions among noise exposed young adults. Occup Environ Med, 61, 899-907.
Seixas, N.S., Neitzel, R., Stover, B., Sheppard, L., Feeney, P., Mills, D., & Kujawa, S. (2012). 10-Year prospective study of noise exposure and hearing damage among construction workers. Occup Environ Med, 69, 643-650.
Sergeyenko, Y., Lall, K., Liberman, M.C., & Kujawa, S. (2013). Age-related cochlear synaptopathy: an early-onset contributor to auditory functional decline. J Neurosci, 33, 13686-13694.
Sininger, Y. (2008). Auditory Neuropathy Spectrum Disorder: Continued challenges and questions. In J. Northern (Ed.), Guidelines for identification and management of infants and young children with Auditory Neuropathy Spectrum Disorder (pp. 9 – 14). Available from www.childrenscolorado.org
Spankovich, C., Le Prell, C.G., Hood, L.J., & Lobarinas, E. (2017). Noise history and auditory function in young adults with and without Type-1 diabetes. Ear Hear, doi: 10.1097/AUD.0000000000000457
Stamper, G.C., & Johnson, T.A. (2015a). Auditory function in normal-hearing, noise-exposed human ears. Ear Hear, 36, 172-184.
Stamper, G.C., & Johnson, T.A. (2015b). Letter to the Editor: Examination of potential sex influences in auditory function in normal-hearing, noise-exposed human ears, Ear Hear, 36, 172-184. Ear Hear, 36(6), 738-40.
Starr, A., Picton, T.W., Sininger, Y., Hood, L.J. & Berlin, C.I. (1996). Auditory neuropathy. Brain, 119 (Pt 3), 741-753.
Starr, A., Sininger, Y.S., & Pratt, H. (2000). The varieties of auditory neuropathy. J Basic Clin Physiol Pharmacol, 11, 215-230.
Subramaniam, M., Henderson, D., & Spongr, V. (1994). The relationship among distortion-product otoacoustic emissions, evoked potential thresholds, and outer hair cells following interrupted noise exposures. Ear Hear, 15, 299-309.
Subramaniam, M., Salvi, R.J., Spongr, V.P., Henderson, D., & Powers, N.L. (1994). Changes in distortion product otoacoustic emissions and outer hair cells following interrupted noise exposures. Hear Res, 74, 204-216.
Sulaiman, A.H., Husain, R., & Seluakumaran, K. (2015). Hearing risk among young personal listening device users: Effects at high-frequency and extended high-frequency audiogram thresholds. J Int Adv Otol, 11, 104-109.
Summers, V., Makashay, M.J., Theodoroff, S.M. & Leek, M.R. (2013). Suprathreshold auditory processing and speech perception in noise: hearing-impaired and normal-hearing listeners. J Am Acad Audiol, 24, 274-292.
Thakur, L., Anand, J.P., & Banerjee, P.K. (2004). Auditory evoked functions in ground crew working in high noise environment of Mumbai airport. Indian J Physiol Pharmacol, 48, 453-460.
Valderrama, J.T., Beach, E.F., Yeend, I., Sharma, M., Van Dun, B., & Dillon, H. (2018). Effects of lifetime noise exposure on the middle-age human auditory brainstem response, tinnitus and speech-in-noise intelligibility. Hear Res, 365, 36-48.
Valero M.D., Burton J.A., Hauser S.N., Hackett T.A., Ramachandran R., & Liberman, M.C. (2017). Noise-induced cochlear synaptopathy in rhesus monkeys (Macaca mulatta). Hear Res, 353, 213-223.
Valero, M.D., Hancock, K.E., & Liberman, M.C. (2016). The middle ear muscle reflex in the diagnosis of cochlear neuropathy. Hear Res, 332, 29-38.
Valero, M.D., Hancock, K.E., Maison, S.F., & Liberman, M.C. (2018). Effects of cochlear synaptopathy on middle-ear muscle reflexes in unanesthetized mice. Hear Res, 363, 109-118.
Viana, L.M., O'Malley, J.T., Burgess, B.J., Jones, D.D., Oliveira, C.A., Santos, F.,…Liberman, M.C. (2015). Cochlear neuropathy in human presbycusis: Confocal analysis of hidden hearing loss in post-mortem tissue. Hear Res, 327, 78-88.
Wilson, R.H., Carnell, C.S., & Cleghorn, A.L. (2007). The Words-in-Noise (WIN) test with multitalker babble and speech-spectrum noise maskers. J Am Acad Audiol, 18, 522-529.
Wilson, R.H., & McArdle, R. (2007). Intra- and inter-session test, retest reliability of the Words-in-Noise (WIN) test. J Am Acad Audiol, 18, 813-825.
Wojtczak, M., Beim, J.A., & Oxenham, A.J. (2017). Weak middle-ear-muscle reflex in humans with noise-induced tinnitus and normal hearing may reflect cochlear synaptopathy. eNeuro, 4.
Wu P.Z., Liberman L.D., Bennett K., de Gruttola V., O'Malley J.T., & Liberman, M.C. (2018). Primary neural degeneration in the human cochlea: Evidence for hidden hearing loss in the aging ear. Neuroscience. doi: 10.1016/j.neuroscience.2018.07.053
Wynne, D.P., Zeng, F.G., Bhatt, S., Michalewski, H.J., Dimitrijevic, A., & Starr, A. (2013). Loudness adaptation accompanying ribbon synapse and auditory nerve disorders. Brain, 136, 1626-1638.
Xu, Z.M., Vinck, B., De Vel, E., & van Cauwenberge, P. (1998). Mechanisms in noise-induced permanent hearing loss: an evoked otoacoustic emission and auditory brainstem response study. J Laryngol Otol, 112, 1154-1161.
Yeend, I., Beach, E.F., Sharma, M., & Dillon, H. (2017). The effects of noise exposure and musical training on suprathreshold auditory processing and speech perception in noise. Hear Res, 353, 224-236.
Zeng, F.G., Kong, Y.Y., Michalewski, H.J., & Starr, A. (2005). Perceptual consequences of disrupted auditory nerve activity. J Neurophysiol, 93, 3050-3063.
Zeng, F.G., Oba, S., Garde, S., Sininger, Y. & Starr, A. (1999). Temporal and speech processing deficits in auditory neuropathy. Neuroreport, 10, 3429-3435.
Citation
Le Prell, C. (2018). 20Q: Hearing science "hide and seek" - can audiologists diagnose hidden hearing loss? AudiologyOnline, Article 24038. Retrieved from www.audiologyonline.com